The most common diseases of the bile and biliary tract—gallstones (cholelithiasis) and inflammation of the gallbladder (cholecystitis), biliary tract (cholangitis), and pancreas (pancreatitis)1—can result in high morbidity and mortality.2 A typical complication is the narrowing (stenosis) or closure (obstruction) of the biliary tract caused by gallstones3 or chronic lesions that form due to gallstones, acute or chronic inflammation of the pancreas or biliary tract, removal of the gallbladder, tumors (benign), or radiotherapy. Bile-duct carcinomas (adenocarcinomas) or carcinoma of the pancreas (pancreatic cancer) can cause malignant strictures, which occur more frequently than benign stenosis.4
Biliary endoprostheses (or biliary stents) were developed in the 1970s to ensure drainage of the bile5 and became an established method over the next decade.6-10 Endoscopic implantation of biliary endoprosthesis does not lead to many complications, and the mortality rate is very low.11,12 However, deposits may accumulate in the stents and result in complete closure, requiring stent replacement.5,13-15 The process of encrustration formation and accumulation inside the stent is still unclear.16 Stent encrustations consist mainly of bacteria, yeast cells, and plant fibers (from food residues). For this analysis, bacterial cultures were prepared from the stent encrustations.4 While the impact of bacteria is known, the influence of factors such as stent material and design is not fully understood.5 This article describes a method used to determine calcium and trace metals using inductively coupled plasma/mass spectrometry (ICP/MS) to investigate the elementary composition of encrustations in clogged biliary endoprostheses.
Materials and methods
Chemicals, standards, and reagents
The following ICP standard solutions were purchased from Merck (Darmstadt, Germany): multielement standard IV containing the elements Ag, Al, B, Ba, Bi, Ca, Cr, Cu, Fe, Ga, In, K, Li, Mg, Mn, Na, Ni, Pb, Sr, Tl, Zn, Cd, and Co, each in a concentration of 1000 mg/L, and single-element standards of Ca, Lu, and Re, each in a concentration of 1000 mg/L. Lutetium was used as the first internal standard (ISTD1) for error correction in the sample introduction, and rhenium as the second internal standard (ISTD2) for correcting vaporizing effects during microwave digestion of the solid samples. ISTD2 was handled like an analyte; the correction of the measurement results was done according to Fleischer et al.17
Microwave digestion, calibration solutions, diluted samples, and rinsing solutions were prepared using Suprapur nitric acid (65%) and Suprapur hydrochloric acid (30%) (Merck) and ultrapure water. Because certified reference material of encrustations of biliary endoprosthesis is not commercially available, and the number and weight (tens of milligrams) of real patient samples is too low to be spiked for several experiments, the researchers prepared a material similar to encrustations of biliary endoprosthesis with a total weight of 50 g containing the following analytes: 0.32 g calcium oxalate (>99.999%) from Sigma-Aldrich (Steinheim, Germany), 1.00 g cholesterol (>95%) from TCI (Eschborn, Germany), 48.68 g high-purity potato starch from Carl Roth (Karlsruhe, Germany), and 1 mL ICP multielement standard IV from Merck. All components were mixed thoroughly in ultrapure water. The final material was dried at 30 °C (86 °F) until a stable weight was reached, and was then pulverized. Table 1 shows the components of the reference material and the expected concentrations.
Table 1 – Composition and concentrations of reference material 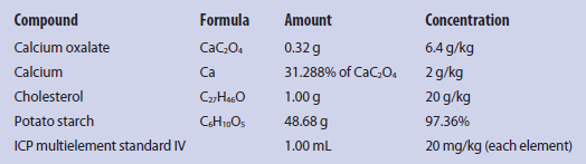
Sample and standard solution preparation
Nitric acid (65%) was used as digestion acid, to which ISTD2 was added with a final concentration of 1 mg/L. After dilution (1:12.5), the concentration of Re was 80 µg/L. Approximately 30 mg of dried and milled sample was weighed and 1 mL of the prepared digestion acid was added. Predigestion was performed in open vessels for 20 minutes at room temperature. One blank sample was included in each digestion run. Microwave digestion was performed using the Mars 5 system with Xpress 10-mL digestion vessels (CEM, KampLintfort, Germany) (see Figure 1). The vessel volume enables the use of low sample weights (up to 30 mg), making it suitable for medical samples.17-20 Magnetic PTFE-coated stirrers (VWR, Darmstadt, Germany) were used for mixing during digestion.
Figure 1 – Microwave digestion turntables and Xpress vessels with the following volumes: a, b) 75 mL; c, d) 25 mL; e, f) 10 mL.
Microwave digestion was carried out with a temperature-time ramp for 20 min to a final temperature of 150 °C (302 °F) and hold time of 20 minutes. The microwave power is dependent on the number of vessels in the device—typical values are 300 W for one to five vessels, 600 W for more than five vessels, and 1200 W for more than 16 vessels. The vessels were cooled to room temperature and uncapped. Ultrapure water (5.75 mL) and digestion solution (0.5 mL) were transferred into a 15-mL plastic tube and mixed. Calibration standards were prepared in nitric acid (8%, v/v) in five concentration levels: calcium—0.101, 0.505, 1.010, 5.050, and 10.100 mg/L; other elements of the multielement standard IV and rhenium—1, 5, 10, 50, and 100 μg/L. All solutions for microwave digestion, calibration, and samples were arranged and stored in polyethylene or polypropylene vessels.
Measurement parameters
Measurements were done using a 7700x ICP-MS (Agilent Technologies, Waldbronn, Germany) with the following parameters: 1550-W radiofrequency power, 10-mm sample depth, 0.65-L/min carrier gas flow, 0.40-L/min dilution gas flow, 0.10-rps nebulizer pump speed, and 13 °C (55.4 °F) spray chamber. Argon was used as plasma, carrier, and dilution gas. In the collision cell, a helium flow was used as follows: 0 mL/min in NoGas tuning mode, and 4.3 mL/min in He tuning mode to prevent polyatomic interferences (see Table 2). Each measurement was performed with three replicates. Sample introduction was done with an ASX-500 autosampler from Cetac (Omaha, NB). For rinsing, two solutions were used: a mixture of nitric acid (5%, v/v) and hydrochloric acid (1%, v/v) (solution 1), and nitric acid (8%, v/v) (solution 2). Rinse time was set to 55 seconds at 0.3 rps of the nebulizer pump (solution 1), followed by 45 seconds at 0.4 rps (solution 2).
Table 2 – ICP/MS analysis parameters (elements, isotopic masses, and tuning mode)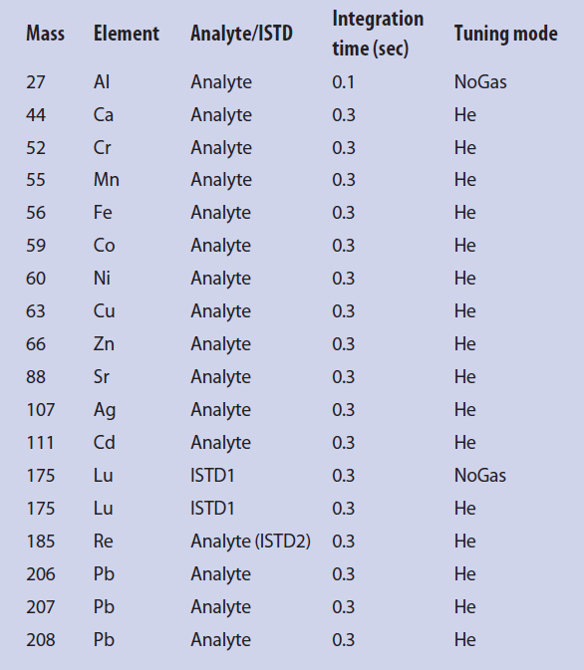
Results
Method validation experiments, including determination of repeatability, recovery rate, within-laboratory precision, method stability, measurement precision, and limits of detection (LOD) and quantification (LOQ), were carried out using the lab-made reference material. Figure 2 shows a typical mass spectrum of a reference sample.
Figure 2 – ICP/MS mass spectrum of a reference sample
Method validation for calcium determination
Repeatability was determined for 27 samples with an expected calcium concentration of 2 g/kg. The average concentration measured was 1.92 g/kg, coefficient of variation (CV) was 6.02%, and average recovery rate was 96% (min. 82%, max. 104%). Within-laboratory precision was determined for seven samples prepared and measured on five consecutive days. The mean values ranged from 1.79 g/kg to 1.97 g/kg and CV values were 2.92%–5.14%. A set of seven samples was used to assess method stability, and the samples were prepared and divided into four sections. One part was immediately measured. The remaining parts were measured on three additional days. Average values ranged from 1.85 g/kg to 2.10 g/kg and CV values were 2.58%–7.20%. One sample was measured nine times to determine measurement precision. The mean value was 1.83 and CV value was 0.85%. Ten blank samples were prepared and measured to ascertain the limits of detection and quantification. In the measurement solutions, values were 0.05 mg/L (LOD) and 0.16 mg/L (LOQ); in the solid samples, they were 22.84 mg/kg (LOD) and 64.78 mg/kg (LOQ). These values were calculated according to Eqs. (1) and (2):
LOD = x̅ + 3∙σ (1)
LOQ = x̅ + 10∙σ (2)
where x̅ is average of 10 blank samples, and σ is standard deviation.
The overall validation results show low CV values with a maximum of 7.2%. Recovery rates were in the range 82–104%. The method developed is thus suitable for the determination of calcium in biliary endoprostheses. Results are shown in Figure 3.
Figure 3 – Results of validation experiments.
Method validation for trace metal determination
The same method was validated for heavy metal determination. Repeatability and recovery rates were determined with the same 27 samples with an expected concentration of 20 mg/kg for each metal. Results of the determination of repeatability, recovery rate, LOD, and LOQ of selected metals are given in Table 3.
Table 3 – Results of the determination of the repeatability, recovery rate (RR), LOD, and LOQ of selected metals 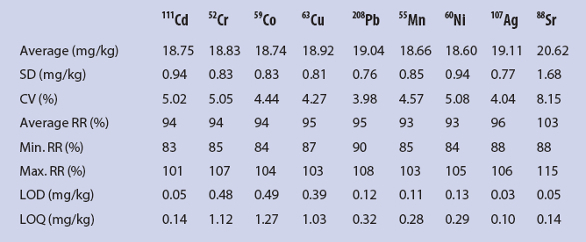
Conclusion
The method presented enables the analysis of calcium and heavy metals in encrustations of clogged biliary endoprosthesis.
References
- Gallagher, T.K.; Parks, R.W. Surgery 2014, 32(12), 635–42.
- Gores, G.J. Curr. Opin. Gastroent. 2008, 24(3), 346–8.
- Caddy, G.R.; Tham, T.C.K. Best Pract. Res. Cl. Ga. 2006, 20(6), 1085–1101.
- Dowidar, N.; Kolmos. H.J. et al. Scand. J. Gastroent. 1991, 26(11), 1137–44.
- Dowidar, N., Kolmos, H.J. et al. Scand. J. Gastroent. 1992, 27(1), 77–80.
- Soehendra, N.; Reynders-Frederix, V. Endoscopy 1980, 12(1), 8–11.
- Cotton, P.B. J. Gastroent. Hepatol. 1990, 5(Suppl. 1), 63–77.
- Huibregtse, K.; Katon, R.M. et al. Endoscopy 1986, 18(4), 133–7.
- Ponchon, T.; Gallez J.-F. et al. Gastrointest. Endosc. 1989, 35(6), 490–8.
- Cotton, P.B.; Forbes, A. et al. Gastrointest. Endosc. 1987, 33(6), 411–2.
- Cowling, M.G.; Adam, A.N. World J. Surg. 2001, 25(3), 355–9.
- Smith, A.C.; Dowsett, J. F. et al. Lancet 1994, 344(8938), 1655–60.
- Dowidar, N. and Moesgaard, F. Scand. J. Gastroenterol. 1991, 26(11), 1132–6.
- Costa, L.; Bracco, P. et al. Biomaterials 2001, 22(23), 3113–19
- Guaglianone, E.; Cardines, R. et al. Microb. Ecol. Health D. 2008, 20(4), 207–9.
- Farnbacher, M.J.; Kraupa, W. et al. J. Med. Eng. Technol. 2013, 37(1), 10–16.
- Fleischer, H.; Vorberg, E. et al. Am. Lab. 2014, 46(6), 16–20.
- Fleischer, H. and Thurow, K. Am. Lab. 2013, 45(8), 6–9.
- Fleischer, H. and Thurow, K. Am. Lab. 2013, 45(9), 6–11.
- Fleischer, H.; Vorberg, E. et al. 5th IMEKO TC19 Symposium on Environmental Instrumentation and Measurement, 2014; pp 94–9.
Heidi Fleischer, Ph.D., is senior scientist, and Kinjal Ramani, M.Sc., and Sergej Bauer are junior scientists from the Institute of Automation, University of Rostock, Richard-Wagner-Strasse 31, 18119 Rostock, Germany; tel.: +49 (0) 381 498 7803; e-mail: [email protected]; www.unirostock.de. Mareike Warkentin, Ph.D., and Detlef Behrend, Ph.D., are from the chair of Materials for Medical Engineering, University of Rostock. Kerstin Thurow, Ph.D., is CEO, Center for Life Science Automation (celisca), University of Rostock. The authors thank the German Federal Ministry of Education and Research for funding the program KMU-innovativ: Medizintechnik, contract number 13GW0082D.