Featured Article
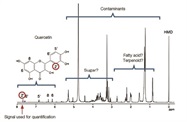
Looking back, NMR spectroscopy has been in use as a research tool and under constant development for almost 80 years. Some principles of magnetic resonance were initially described by Isidor Isaac Rabi of Columbia University in 1938. With further fundamental research being done in the late 1930s and early ’40s, the official birth of the technique is generally accepted to have been in 1946 and is attributed to Edward M. Purcell and Felix Bloch. Both were honored with the Nobel Prize for Physics in 1952 for the discovery of NMR spectroscopy. Dr. Ray Freeman, one of the pioneers of NMR applications technology, has created a resource for further reading on the history of both the science and development of NMR technology.1
The technique has undergone significant developments in hardware, probe design, and software since that time—developments that have unlocked new applications. It has proven to be particularly beneficial in many of the applied industry sectors where it is now an established part of the analyst’s toolbox (pharmaceuticals and biopharmaceuticals, food, environmental, polymer science, and proteomics, for example). A core reason for its success in these markets is that NMR offers unparalleled breadth of information about the sample, including detailed structural information. Moreover, it provides straightforward method development, allows rapid throughput without loss of the sample, and, importantly, is a direct quantitative method with no requirement for response factors or calibration curves.
Overcoming early technical barriers
By the end of the 1950s, technical limitations were putting a brake on the application of NMR. Up to this point, NMR data was acquired by scanning a sample across a broad range of radiofrequencies, in a repeating sequence, until enough signal was obtained to be useful. So-called “continuous wave” NMR (CW-NMR) was a time-consuming process, taking several minutes to scan each frequency. Hundreds, or even thousands, of scans were required to resolve a single sample.
This changed in 1969 with the first demonstration of an FT-NMR spectrometer. This involved collecting scans in parallel and using the Fourier transformation to convert the resulting data so that it retained consistency with CW-NMR. The first results were published by Ernst and Anderson in 1966,2 and, by 1972, a fully developed commercial system was on the market. The shift from CW-NMR to the inherently digital FT-NMR made necessary the inclusion of an integrated digital computer as part of the NMR spectrometer. The introduction of multidimensional NMR greatly increased the demands on the computer, necessitating a move to high-power graphics computer workstations in the early 1990s. JEOL was a pioneer in creating powerful NMR software solutions to support this important transition. The development of FT and multidimensional NMR from the mid-’60s through the mid-’80s ultimately led to the 1992 Nobel Prize in Chemistry being awarded to Richard R. Ernst.
With FT-NMR established as the standard NMR approach, together with the benefits derived from replacing iron-core magnets with superconducting magnets, the practical limits of previous NMR systems were removed. Increasing a magnet’s field strength from 200 MHz to >500 MHz to boost sensitivity and dispersion was simply a matter of scaling up existing technology, while improvements in computing technology, software, and programmed pulse sequences allowed for more efficient use of the magnet hardware. In 2015, JEOL worked with the National Institute for Materials Science (NIMS), RIKEN, and Kobe Steel in Japan to develop the first ultrahigh-field 1030-MHz (24.2T) magnet that uses a high-temperature superconducting insert to boost the magnetic field beyond traditional magnets.
The role of probe technology
The NMR probe is critical for determining the quality of an experiment. As the demand for heightened sensitivity continues, probes are today a key area of innovation, as they are the first step in signal detection and also impact throughput—if a probe is more sensitive, you can collect more data in a shorter timeframe. The long tradition of NMR probe flexibility and ease of use began in 1978 with the Omni probe, and was continued by the invention and introduction by JEOL of automatic probe tuning in 1985—a key piece of technology in NMR. Probe auto-tuning is an industry standard today.
The idea of sample spinning to average magnetic field effects was first suggested by Felix Bloch to Jim Arnold as they struggled to approach high resolution in liquids NMR. While sample spinning is of declining need in liquids NMR today, very high-speed sample spinning provides a leg on which solids NMR stands. Spinning speed for solid samples has continued to increase since it was first introduced by Andrew3 and Lowe4 in the late 1950s. High-speed spinning probes had a progressive effect on solid-state NMR and provide improved sensitivity while enhancing resolution. JEOL’s 0.75-mm solid-state NMR probe spins the sample at 120 kHz (7.2 million rpm); it was the world’s fastest spinning probe when launched in 2015.
Cold probes were first introduced to increase the sensitivity of the probe coil and reduce the level of thermal noise generated by electronic circuits and components of the receiver. On average, cold probes improve signal-to-noise ratio three- or fourfold. Cold probes began with helium cooled chillers; however, as a lower-cost and easier-to-maintain alternative, nitrogen cooled probes are now also being utilized.
A more recent probe development addresses the growing need for better analysis of fluorine-containing samples. Currently, more than 200 marketed medicines contain fluorine atoms in their structure. These compounds span a range of therapeutic classes: anticancer drugs, antifungal agents, and nonsteroidal anti-inflammatory drugs (NSAIDs), for example. Fluorine is also now commonly found in illicit and illegal synthetic drugs, including synthetic cannabinoids and psychedelic phenethylamines. Therefore, 19F NMR spectroscopy has become an important tool for their analysis.
Previously, widespread use of a fluorine probe in NMR was not well-implemented as it required specialized hardware to allow the uniform excitation of the broad 19F chemical shift range and was limited in capability. However, with modern hardware configurations on new NMR spectrometers, 19F NMR has been shown to be a useful tool for routine structure elucidation, and modern NMR probes often allow easy, straightforward use of 19F NMR or 1H and 13C NMR, but not all three at the same time. The ROYAL HFX NMR Probe (Figure 1) offers the ability to manipulate the 1H, 19F, and 13C spins simultaneously without the typical loss in performance associated with traditional NMR probes designed for proton–fluorine NMR spectroscopy. The probe’s patent-pending magnetic coupling technology provides high-resolution NMR in HFX or HX mode with no performance loss in HX mode relative to a dedicated HX-only NMR probe.5
Figure 1 – Illustration of magnetic coupling technology used as a switch between single-tune and dual-tune modes in JEOL’s ROYAL HFX Probe. Emerging NMR applications: the food industry
Food characterization and traceability have becoming increasingly important due to food fraud scandals and the changing attitudes of consumers to the origin and makeup of what they eat. Regulatory bodies have also intensified the scrutiny of all producers, manufacturers, and sellers of food such that food testing labs are utilizing a new suite of technology to analyze and monitor the quality of our food. NMR is an ideal candidate for food testing—samples can often be processed in their original state, without the need for complex sample prep. It is also a rapid screening technique, with measurements performed in minutes.
An example of a product that can be analyzed with the help of NMR is blue cheese. Production methods, raw ingredients, and local customs have led to a wide range of types of blue cheese available. In such cases, authenticity and the scope for adulteration or “passing off” a less expensive product as a higher-value one are significant. The type and amount of amino acids, characteristic odor components, and detailed observation of the blue mold are all relevant and can be analyzed using, for example, scanning electron microscopy, dedicated amino analyzers, GC/MS, and NMR. Work has shown NMR resonances corresponding to the amino acid “signature” of a cheese can be observed and quantified.
The growing appeal of quantitative NMR
Work completed as part of a collaboration between JEOL and the National Metrology Institute of Japan6 improved an existing 1H NMR method to enable accurate and rapid quantification of analytes derived from natural sources when it is difficult to obtain reference material for quantification. The subject of this project was Tartary buckwheat, a foodstuff that contains large amounts of rutin as a functional flavonoid. Quercetin, a flavonoid, can be detected as a degradation product of rutin in samples because there are rutin-degrading enzymes in Tartary buckwheat noodles. The amount of quercetin found in a sample of noodles was quantified using hexamethyldisilane (HMD) as an internal reference standard. It was determined that the content of quercetin was 1.58 ± 0.14 mg per gram of Tartary buckwheat. Figure 27 shows the methanol extract 1H NMR spectrum.
Figure 2 – qNMR allows for quick and accurate quantification of analytes from natural sources when obtaining a reference material is difficult.Looking to the future
The pace of NMR hardware developments continues to accelerate and recent innovations in NMR probes and the establishment of new applications mean that NMR is set to increase in importance. A shift to real-time analysis and an increasing demand for rapid screening techniques confirm NMR as one of the key techniques for most analytical chemists. Quantitative applications are adding a further dimension to NMR application in several industries where the technique has not previously been routine. Furthermore, as sensitivity continues to increase, and new probe development advances, NMR has become a “go-to” method for those working in life sciences and ’omics, as well as biopharmaceuticals and small-molecule drug development and manufacture.
A technique with its roots in the 1930s has matured to become a mainstream analytical tool in 2018, with an exciting future ahead.
References
- http://www.ray-freeman.org/index.cgi
- Ernst, R.R. and Anderson, W.A. Rev. of Scientific Instruments 1966, 37, 93.
- Andrew, E.R.; Bradbury, A. et al. Nature 1958, 182, 4650.
- Lowe, I.J. Phys. Rev. Lett. 1959, 2(7), 285–7.
- https://www.jeolusa.com/hfxinfokit
- Ihara, T. and Saito, T. Realization of an innovative metrological traceability using the quantitative NMR method. National Metrology Institute of Japan, National Institute of Advanced Industrial Science and Technology, 2012.
- Sugimoto, N. and Tada, A.Rapid Quantification of Quercetin in Tartary Buckwheat Noodle by Quantitative NMR. National Institutes of Health, 2010.
Michael H. Frey is analytical instruments product manager, and Ron Crouch is NMR applications consultant, JEOL USA, Inc., 11 Dearborn Rd., Peabody, MA 01960, U.S.A.; tel.: 978-535-5900; e-mail: [email protected]; www.jeolusa.com