Featured Article
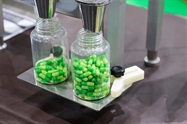
Solvents and other volatile organic compounds are used extensively in the manufacture of a diverse range of consumer goods, including food packaging and pharmaceutical products. These substances play a variety of important roles, from the solvents used to synthesize pharmaceutical ingredients to those that ensure packaging or tablet coating materials have the necessary physical characteristics to function effectively. Additionally, solvent-based dyes and inks are used to convey batch information or make products visually appealing to consumers.
Despite measures to remove harmful volatile compounds from the final product throughout manufacturing and purification processes, these substances cannot always be eliminated, potentially putting consumer health at risk. Even when not harmful, the leaching of volatile compounds present in food contact materials into foodstuffs can also affect product taste, smell, and appearance.
Regulatory measures are in place for the determination of residual solvents to ensure pharmaceutical products or food packaging materials do not contain unacceptable levels of residual volatile compounds used in manufacturing processes. In the United States, a migration limit of 50 parts per million (ppm) is applicable for residual solvents in food products.1 Meanwhile, in the European Union, there are robust regulations to protect the safety of food contact materials,2 and the precise quantitation of residual solvents in flexible food packaging is regulated through well-established standards such as European Standard EN 13628-1:2002.3 Similarly, for the control of residual solvents in pharmaceutical products, the International Conference on Harmonisation of Technical Requirements for Registration of Pharmaceuticals for Human Use4 refers to United States Pharmacopeia (USP) Method <467> for the analysis of volatile organic impurities.5 To support these requirements and ensure food and pharmaceutical products are safe for consumption, reliable methods for residual solvent analysis are essential.
Advances in headspace sampling simplify and enhance residual solvent analysis
Gas chromatography (GC) is ideally suited for the analysis of volatile organic impurities like residual solvents due to their relatively low boiling points and high thermal stability. However, the analysis of volatile impurities in solid matrices, like polymers or powder samples, can be challenging using traditional liquid injection techniques and involve time-consuming sample preparation and extraction steps.
Liquid injection requires samples to be extracted with solvents prior to analysis, potentially resulting in the extraction of nonvolatile compounds that can accumulate in the GC injector ports, necessitating regular inlet liner replacement. This can increase system maintenance requirements, analysis costs, and instrument downtime, ultimately impacting laboratory efficiency and productivity.
Static headspace sampling techniques offer an alternative to liquid injection for volatile compounds, enabling solventless extraction from complex liquid and solid matrices without the need for time-consuming sample preparation steps. Consequently, static headspace sampling coupled with gas chromatography (HS-GC) and flame ionization detection (FID) or mass spectrometry (MS) is the method of choice for residual solvent determination in food packaging materials and pharmaceutical products. Advances in static headspace autosampling technology are helping scientists working across a range of fields increase throughput in residual solvent analysis in a regulatory-compliant manner.
Improving measurement reliability with advanced headspace sampling technologies
The analysis techniques used to assess residual solvent content in food and pharmaceutical products must deliver reliable and consistent measurements that meet standard method requirements. Static headspace autosamplers, based on valve-and-loop technology for sample injection, are recommended in highly regulated environments as the best approach for reliable and precise results. Ongoing advances are enhancing the capabilities of these systems to support the highest levels of injection repeatability and operational robustness.
Innovative design features in modern valve-and-loop HS-GC systems are helping analysts achieve high performance when it comes to routine residual solvent analysis. For example, pneumatic circuits that directly connect the heated valve of the headspace autosampler to the GC column significantly reduce the sample path and support high system inertness. This enables optimal sample integrity during transfer, improves the recovery of less volatile compounds, and ensures highly precise sample introduction with excellent peak area repeatability. Continuous purging of the sample path eliminates the risk of contamination and carryover of high-boiling-point solvents, and supports the collection of high-quality, reliable data on a routine basis.
To demonstrate the effectiveness of modern HS-GC systems for residual solvent analysis in pharmaceutical products according to USP Method <467>, solutions of aspirin and, separately, paracetamol pain relief tablets were spiked with solvent solutions and analyzed using the Thermo Scientific TriPlus 500 GC Headspace Autosampler. This method also sets concentration limits for residual solvents based on their toxicities. Class 1 solvents are defined as those with unacceptable toxicities, Class 2 solvents have less severe toxicity, and Class 3 solvents have low toxicity.4,5Figure 1 highlights chromatograms obtained for Class 1 system suitability solutions for water-soluble and water-insoluble products. The signal-to-noise (S/N) ratios met and exceeded the method performance criteria for USP Method <467>.
Figure 1 – Peak-to-peak signal-to-noise ratios for Class 1 system suitability solutions in (a) water-soluble and (b) water-insoluble pharmaceutical products.
System repeatability was assessed using 18 consecutive injections of standard solutions diluted in water or dimethyl sulfoxide (DMSO) according to the USP procedure for water-soluble or water-insoluble products, respectively. Peak area relative standard deviation (%RSD) showed average values <3% for all residual solvent classes when water and DMSO were used as diluent, with the majority of the compounds spanning 0.4–1.5 %RSD, confirming the high precision of the sampling process.
To further evaluate the performance of the HS-GC system for USP Method <467>, response linearity was also assessed. For stock solutions of Class 1, Class 2A, and Class 2B residual solvents, four calibration standards were assessed at 12.5%, 25%, 50%, and 100% of the concentration limit. Each sample was prepared and analyzed in triplicate. Across all the residual solvents, an average coefficient of determination (R2) of 0.998 was determined, with a %RSD of the response factors across the calibration levels of less than 8%, highlighting the excellent linearity of the response. The linearity of representative residual solvents from each of the three solvent classes is shown in Figure 2.
Figure 2 – Calibration curves for benzene, methylcyclohexane, and trichloroethane obtained by HS-GC-FID.
Reducing time by eliminating carryover
High-boiling-point solvents used to dissolve samples for headspace analysis may be detected at trace levels in the next sample. A key challenge is dealing with possible carryover, which can overestimate concentrations in quantitative results. To avoid false results, multiple blank runs typically need to be included in the sequence to ensure negligible residual area counts for the solvents to be quantified. However, running blanks can significantly extend sequences.
Using a direct column connection to the heated valve of the headspace autosampler enables more efficient heating of the shorter sample path while reducing dead volumes by bypassing the GC injector. These features, along with the continuous purging of the sample path, ensure almost no carryover of high-boiling-point solvents.
Overcoming matrix challenges using multiple headspace extraction
Static headspace sampling is a very effective technique for volatiles analysis in solid and liquid samples. However, for quantitative analysis, it relies on the preparation of homogeneous samples and standards. When handling nonreproducible matrices, such as those associated with some food packaging materials, accurate and reproducible quantitative analyses can be more challenging.
Static headspace with multiple headspace extraction (MHE) offers an effective quantitative approach when matrix-matched calibration reference materials are not available. MHE methods allow the extrapolation of the peak area representative of the total analyte content in a liquid or solid matrix, through multiple headspace injections from the same sample. The amount of analyte can then be determined by directly comparing the peak area responses to external standards analyzed in a similar way, but without matrix.
Figure 3 – MHE linearity for ethyl acetate in (A) sliced salami lid and (B) sliced salami plastic tray, and for (C) ethanol and (D) acetone in salad wrap.
Figure 3 shows calibration curves for ethyl acetate, ethanol, and acetone in various polymer-based food packaging matrices, obtained by MHE. The linearity of the MHE calibration method was assessed by plotting the natural logarithm of the peak areas against the number of headspace cycles (n = 4). The linearity (R2 ≥0.995) exceeds the EN 13628-1:2002 standard method requirements (R2 ≥0.98), demonstrating the suitability of the system for residual solvent quantitation in food packaging.
Untargeted residue identification using advanced microfluidics and dual-detector configurations
Many volatile organic compounds can potentially be released as a result of food packaging manufacturing processes, including those used for printing inks and possible degradation reactions. These compounds may be present at different levels in the final product. To support robust and reliable analyses, the analytical methods used must be capable of accurately identifying these contaminants.
Coeluting and untargeted analytes are challenging to identify using FID detection alone, which uses the retention time of reference standards for compound identification. Modern HS-GC systems are helping to improve confidence in analyte identification by offering a combination of FID and single-quadrupole MS detection. Advanced microfluidic connectors allow analysts to efficiently split the carrier gas flow from the GC column at a specific ratio between two detectors, while ensuring no dead volumes and high sample path inertness. Full-scan MS data can then be used to search against spectral libraries to confirm the identity of detected impurities.
Figure 4 compares the retention times for residual solvent standards obtained by FID and single-quadrupole MS, with the carrier gas flow split 1:1. The strong correlation in retention times between the two detection techniques highlights the robust performance of the microfluidic connection, and demonstrates how an advanced HS-GC system may use a FID/MS dual-detector configuration for combined quantitation and confirmation of co-eluted or unexpected compounds.
Figure 4 – FID and full-scan total ion current (TIC) traces for a residual solvent reference standard.
Conclusion
Advances in static headspace autosampler technology are simplifying the analysis of volatile organic compounds by eliminating time-consuming sample preparation procedures. Improvements in the design of modern valve-and-loop headspace autosamplers featuring direct column connection and precise pneumatic control are pushing performance limits to new levels, increasing injection repeatability, maximizing sample integrity during transfer, and eliminating carryover. By extending the analytical power of GC-MS/FID, modern systems are helping manufacturers meet and exceed regulatory requirements for residual solvent analysis to support the confident identification and quantitation of potentially harmful impurities.
References
- Title 21, Code of Federal Regulation, Direct Additive Part, 170.3; Indirect Additive, Part 174–179.
- Food Contact Materials—Regulation (EC) No 1935/2004—European Implementation Assessment Study, May 2016.
- EN 13628-1:2002 Packaging. Flexible packaging material. Determination of residual solvents by static headspace gas chromatography—Part 1: Absolute methods.
- Impurities: Guideline for Residual Solvents Q3C(R7), ICH Harmonised Guideline, International Council for Harmonisation of Technical Requirements for Pharmaceuticals for Human Use, 2018.
- General Chapter USP <467> Organic Volatile Impurities, Chemical Tests, United States Pharmacopeia, 2012.
Daniela Cavagnino is product marketing manager, Gas Chromatography, Chromatography and Mass Spectrometry, Thermo Fisher Scientific; e-mail: [email protected]; www.thermofisher.com