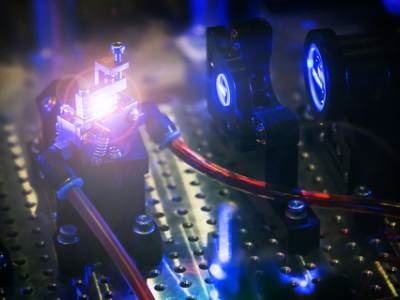
Optical spectrometry is well into a new rapid innovation phase powered by advances in understanding the physics of light combined with elegant engineering leading to useful products. Light is being sculpted by advances in lasers and photonics chips that can couple electronics and optics on a small solid-state device. The demand side is driven by life science research fueled by an insatiable demand for disease and drug discovery for improving health, plus opportunities for novel nanomaterials.
The products are much more than legacy spectrometers such as UV-VIS, IR, and Raman, most of which use refraction, gratings or filters to select and measure intensity of electromagnetic radiation. Physicists are having success with showing that light is more complex than simple frequency and polarization. Light has angular momentum, which must not be destroyed. In the quantum world, there is spin. Photons can interact or entangle with other photons and matter to produce polaritons and other quantum constructs promising new synthetic routes for molecules and efficient renewable energy.
Wave-particle duality
Electromagnetic radiation (light) has a dual character of particles that often behave as waves. Waves have magical properties if one is clever in designing for them. A device developed by a team at IMAC (Interuniversity Microelectronics Center, Belgium) described a light source array for lens free fluorescence imaging.1 A chip-based interference pattern creates a 2-D grid of illumination spots that greatly increases throughput of fluorescence microscopes. But no lens is required. The integrated electronic circuits on the chip also recreates the image. Ten chip devices can fit onto the microscope table, which improves throughput by a similar factor.
Fluorescence microscopy experiments often use chemical fluorophores as labels to aid detection. The labels may introduce artifacts that may lead to ambiguous results. Photobleaching is also a problem with imaging living organisms. A team at the University of Queensland (Australia), for example, has created a quantum microscope for Raman microscopy that enables imaging of live sample—such as yeast—at low light intensity, which avoids photobleaching and sample death.2 The key is illuminating the sample with quantum correlated photons.
In quantum correlation microscopy, the lead photon correlates in space/time with the trailing photon. This fixed time correlation can be used to reduce random noise. Thus, images can be obtained with much lower photon flux, enabling direct, label free detection of various Raman bands in biological structures. If correlation of two photons is good, what about three?
Metamaterials
Metamaterials consist of arrays of dielectric nanostructures that have unique properties, such as high sensitivity and Q factor, but are also CMOS-compatible. These advantages are crucial in designing portable point-of-care or wearable devices.3 In practice, metamaterials respond to changes in surface refractive index (n). For example, researchers have recently described the binding of an anti-mouse antibody to an immobilized mouse IgG with a detection limit of ~770 fM. Similar biosensors for point-of-care diagnostics for diabetes and multiple sclerosis are development targets.
Spectroscopic catalysis
Coherent states of light and matter can be used to direct synthetic chemistry to yield products that are entropically or thermally unfavored by conventional chemical synthetic routes. The ability to selectively control energy and charge in a reaction can catalyze formation of unique products. For example, photovoltaics materials (PV) respond to light, which produces structures that can reversibly produce charge separation and hence electricity.
Research in photovoltaics is delivering startling advances. Recently, researchers at the Fraunhofer Institute for Solar Energy (Germany) demonstrated a conversion efficiency of 68.9% for an ultra-thin gallium arsenide flexible film. The light source is an 858-nm laser.4
Additionally, a session at the Fall 2021 ACS meeting focused on polaritons for catalysis using a range of spectroscopies, including vibrational and electronic and pulse EPR (electron paramagnetic resonance) to induce and control chemical reactions.
Photoacoustic imaging
Photoacoustic (PA) imaging uses pulses of non-ionizing laser light to illuminate a target tissue. The tissue is heated rapidly which produces transient thermoelectric expansion which then generates ultrasonic signals in the MHz range. These are processed for the image. Acoustic waves are not scattered as badly as electromagnetic waves. This improves image quality in deep tissue.
High resolution and fast Raman microscopy
Until recently, the diffraction limit of light has limited spatial resolution of optical imaging in Raman microscopy. Surface enhanced Raman (SERS) has improved the signal strength of Raman vibrations, often by a million-fold. Alexei Sokolov of Texas A&M University and his team of colleagues use a metalized scanning tip similar to an Atomic Force Microscopy tip which acts like a “plasmonic nano-antenna” to generates a Raman spectroscopic signal with nanometer spatial resolution.5 The tip has enabled reading the sequence of single nucleic acid fragments.6
The A&M team also compared the technique's improvements over the state-of-the-art which includes Spontaneous Raman micro spectroscopy which Sokolov characterizes as experimentally easy, but much slower. The coherence-based technique is orders of magnitude more sensitive and therefore faster and is particularly useful in molecular mapping. This technique, when combined with tip-enhanced detection, should be useful in making molecular spectroscopic measurements of small targets including single virus particles and other pathogens.
Micro spectrophotometers
Miniaturization is one of the obvious signs of a mature technology. For example, fluorescence spectrometers circa 1970 were large desktop instruments with analog output. Most were shared at department level. Fast forward to 2021, Ibsen Photonics introduced an ultra-compact fluorescent spectrometer that covers the range of 360 to 830 nm with 6 nm resolution and a feature size of ~ 2 cm, hence the trade name “PEBBLE.”7 The CMOS detector contains 256 pixels. The optical grating has several advantages compared to a filter stack. Filters generally have a wider band pass than even the small grating of PEBBLE. This reduces cross talk between fluorophores, improving quantitative accuracy. Plus, with the grating, one can measure fluorescence at several wavelengths simultaneously, which is useful in applications such as cell sorting, where the sort function may respond differently to Label 1, 2 and 3, in contrast to Label 1 and 2 but not 3.
New lens design
Glass is the conventional material for construction of optical lenses based upon refraction. Scientists at the University of Utah have demonstrated a thin (10 μm) plastic lens for imaging in the IR from 8000 to 12,000 nm.8 The lens technology is called Multilevel Diffractive Lens (MDL). With appropriate design, the MDL can correct for image aberrations. Plus, since MDLs are thin, light loss due to optical absorbance is small. The lens is fabricated by micro imprint lithography. The pattern is an array of small scattering units which provide a local phase shift. This gives a field of view of 35o and angular resolution of 0.013o. This technology is being commercialized by Oblate Optics Inc.
Multiphoton spectroscopy
A team from the Georgia Institute of Technology reports a further improvement in image quality by reducing background using a novel two-pulse illumination scheme to irradiate silica nanoparticles with optically modulable dyes in vivo and in real time.9 Dual photon irradiation greatly reduces the signal from surrounding chromophores surrounding the target. This improves the contrast, image quality and frame rate.
Quantum cascade lasers
Quantum Cascade lasers are tunable and powerful, which is useful for mid IR analysis of gases. For example, Aerodyne Research, Inc. has developed an instrument using laser light from two thermoelectrically cooled quantum cascade lasers selected for their stability and high-power output to measure NH3 at 967 cm-1, NO2 at 1066 cm-1, HNO3 and H2CO at1722 cm-1 and NO at 1909 cm-1. Detection limits are in the range of 10 to 100 ppm with a 210 m folded gas cell.10
3-D imaging
Over the last decade, a variety of super resolution imaging techniques for studying single molecule locations in 3-D biospecimens have been reported.
Recently, a team at the National Institutes of Science and Technology (NIST) developed a protocol to locate point sources in 3-D by calibrating the optics of an ordinary fluorescence microscope.11 One needs only a standard sample to measure the effects of the aberrations and construct the correction, which is applied to the image of the sample. This procedure provides an axial (z) precision of 25 nm over a range of 10 μm. The lateral (x,y) precision is 1 nm over 250 μm. Plus, the imaging frequency is as fast 90 Hz. The research team suggests scientists could apply the calibration technology to existing microscopes without adding equipment.
Holographic Raman microscopy uses surface enhanced Raman scattering from individual cells illuminated with individual photons traversing a grating in a 2-D shearing interferometer to create four image copies that are recombined on camera.12 A slight movement of the grating produces a phase derivative of the four target images. Each of the four image copies are slightly out of phase with the others. The signals are integrated to obtain the original phase and image, which would allow scientists to use nanoparticles to image live cells and 3-D tissue, for example.
Perhaps the most elegant design is the use of stacked solid-state z-splitters to provide 3, 6 or 9 simultaneous image slices along the z axis.13 Typical delta z is 20 to 100 μm. Scientists working at Boston University have developed a 3-D deconvolution /re-convolution algorithm that significantly improves image quality. The researchers note that with the combination of the multifocal image technology and EV-3D deconvolution, they can easily and rapidly record multiple focal planes with a single camera. This enables high contrast functional imaging of complex specimens, such as a mouse brain.
References
- Kouznetsov, B. et al, "Revival and Expansion of the Theory of Coherent Lattices." Physical Review Letters, v 125 #18 (2020). www.doi.org/10.1103/physrevlett.125.184101
- Casacio, C. A. et al, "Quantum-enhanced nonlinear microscopy." Nature, v 594 pg. 201-206 (2021). https://doi.org/10.1038/s41586-021-03528-w
- Karawdeniya, Buddini et al. Australian National University, “Dielectric metasurfaces as refractive index sensors: Towards high-sensitivity label-free optical sensing.” ACS Fall 2021 meeting.
- Photonics Spectra Sept 2021 pg. 30
- Deckert, V. et al, “Enhancement to traditional spectroscopic techniques could lead to the chemical mapping of pathogen, aiding in diagnostics and drug development.” Biophotonics, Sept./Oct. 2021 pg. 33 &ff.
- He, Z. et al, "Tip-enhanced Raman Imaging of single -stranded DNA with single base resolution.” J Am Chem Soc, v 141 #2 pg. 753-757 (2018). https://doi.org/10.1021/jacs.8b11506
- www.ibsen.com
- Meem, M. et al, "Broadband lightweight flat lenses for long-wave infrared imaging." PNAS, v 116 #43 (2019). www.doi.org/10.1073/pnas.1908447116
- Md Shariful Islam, et al, Georgia Institute of Technology. “Background-free modulated photoacoustic Imaging” virtual lecture. American Chemical Society meeting, August 22, 2021.
- www.aerodyne.com
- Copeland, C. R. et al, "Accurate localization microscopy by intrinsic aberration calibration." Nature Communications, v 12 (2021). www.doi.org/10.1038/s41467-021-23419-y
- Liebel, M. et al, "Surface-enhanced Raman scattering holography." Nature Nanotehnology, v 15 pg. 1005-1011 (2020). www.doi.org/10.1038/s41565-020-0771-9
- Xiao, S. et al, "High-contrast multifocus microscopy with a single camera and z-splitter prism." Optica, v 7 #11 pg. 1477-1486 (2020). https://doi.org/10.1364/OPTICA.404678
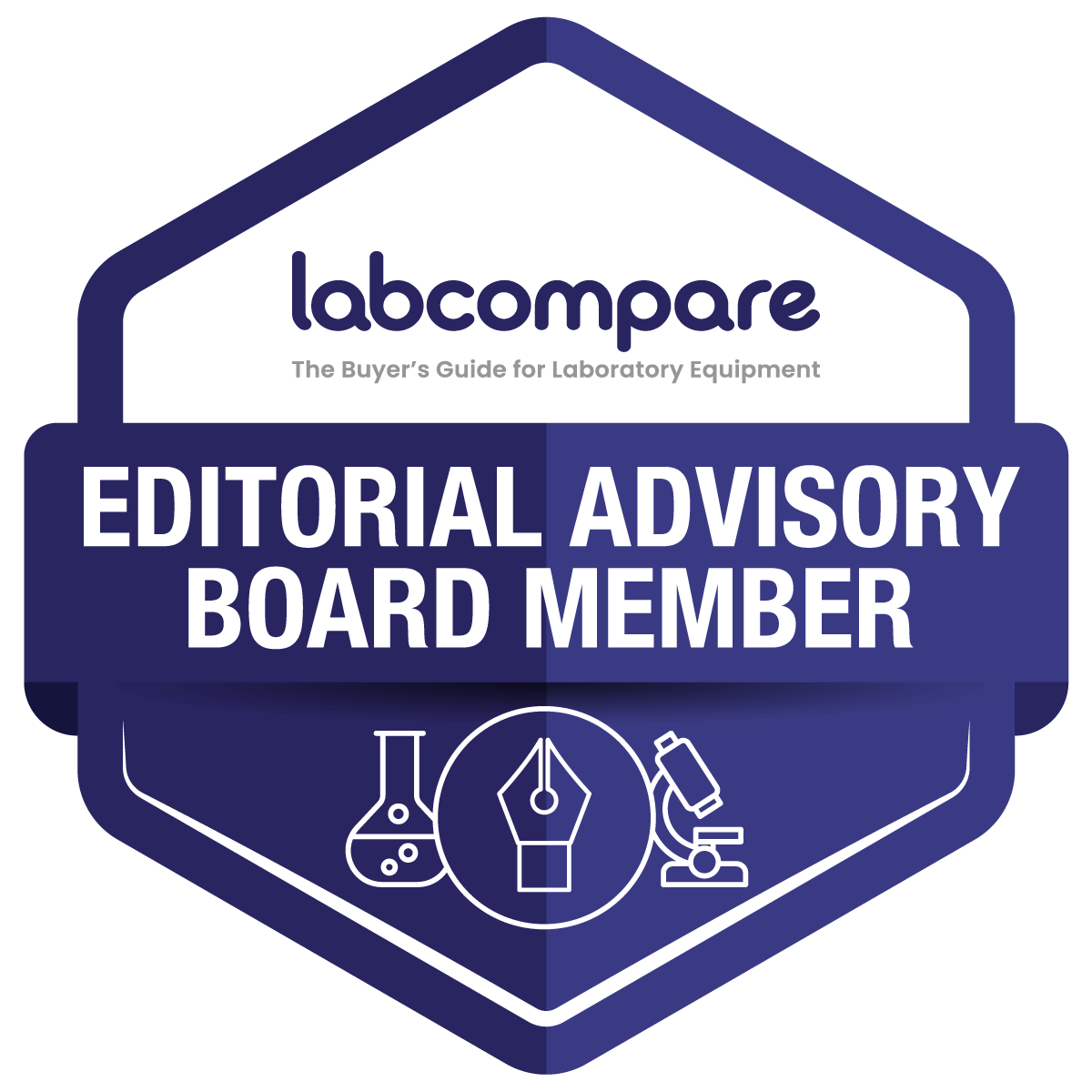
Part II of this article, publishing on March 16, 2022, will discuss the applications that have taken advantage of these advanced optical spectroscopy methods.
About the Author: Robert Stevenson, Ph.D., is a member of Labcompare's Editorial Advisory Board and Editor Emeritus of Labcompare and American Laboratory. He has been involved in developing instrumentation and enabled applications since the late 1960's at firms including Varian, Altex, Bio-Rad, and TosoHaas. Stevenson has been a regular contributor to American Laboratory since its founding in 1968. He has authored more than 450 papers on new technology and applications.