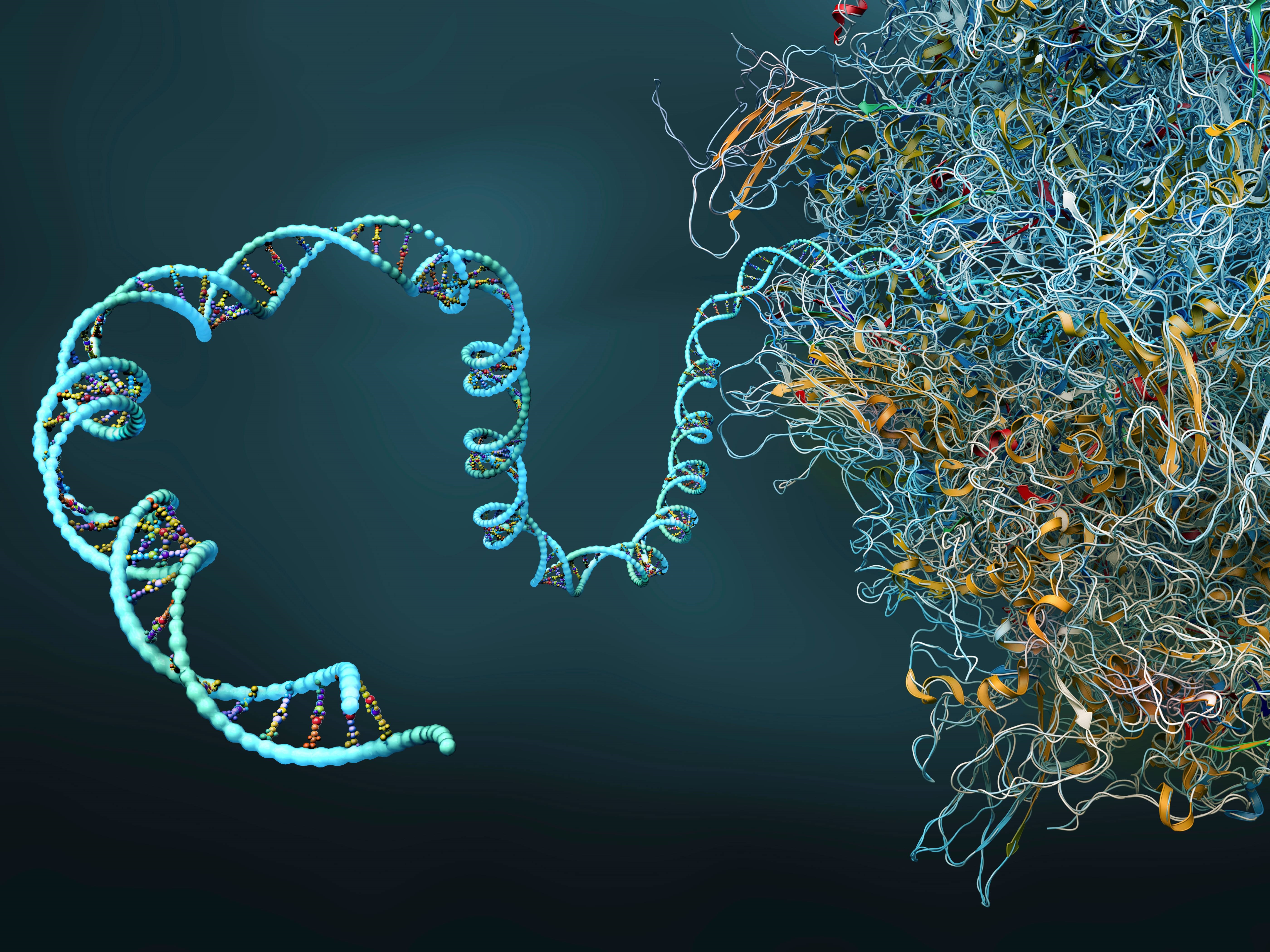
by Josien Levenga and Andrew Riching, PerkinElmer’s Horizon Discovery
From RNA interference (RNAi) to CRISPR, there are several methods that researchers can use to manipulate gene function, each with its own strengths and weaknesses. Orthogonal validation—the synergistic use of different methods—makes genetic perturbation studies more robust. Utilizing complementary methods, including RNAi and CRISPR-knockout, -interference and -activation, enables researchers to have confidence in their results.
Gene Disruption Technologies at a Glance: RNAi vs. CRISPR
Historically, genetic manipulation has been achieved with RNA interference (RNAi) to knockdown a gene of interest, while overexpression was obtained by plasmid-based overexpression of the open reading frame (ORF) of the gene of interest. More recently, the discovery of the clustered regularly interspaced short palindromic repeats (CRISPR) system has greatly expanded the toolbox for studying gene function. These parallel tools to engender similar protein modulation effects as RNAi or ORF overexpression not only enable scientists to cross-validate their results, but also study genes in different contexts. For example, RNAi can be used to suppress messenger RNA (mRNA)/protein expression while CRISPR can be utilized to knock out a gene. These approaches range from transient effects in the case of RNAi knockdown to heritable, permanent loss of gene expression with CRISPR knockout (CRISPRko). In addition to studying a single gene, these tools can be leveraged for whole genome or targeted genetic screens to study transcriptional changes after specific interventions.
RNAi
RNAi is a process by which mRNA is targeted and inhibited, resulting in protein knockdown. Endogenous non-coding RNAs called microRNAs (miRNAs) natively regulate gene expression. miRNAs bind to 3’ untranslated regions (UTRs) of mRNAs via imperfect base pairing and thus can regulate expression of potentially hundreds to thousands of genes. miRNA binding to target mRNA regulates translation through different processes, for example, by destabilizing the mRNA through shortening of its poly(A) tail.
Small interfering RNAs (siRNAs) are 21-23nt, synthetic double-stranded RNA molecules that also utilize the miRNA-based mRNA targeting system. Compared to miRNAs, siRNAs achieve higher specificity due to perfect base pairing to the target mRNA. Delivery of siRNA results in transient gene knockdown; however, more persistent gene knockdown can also be achieved using an expressed RNAi delivery system encoding short hairpin RNAs (shRNAs). Plasmids carrying shRNAs can be transiently transfected or virally packaged and delivered to cells resulting in stable integration into the genome. When stably integrated into the genome, shRNAs create continued knockdown of the targeted protein. Additionally, shRNAs can be expressed from a tissue-specific or inducible promoter, enabling more specific transcriptional control. Since shRNAs are actively transcribed by the cell, they utilize the miRNA processing pathway to generate functional siRNA.
CRISPR
The CRISPR gene editing system, first described in 2012, was adapted from an immune defense system found in bacteria. Using this approach, a CRISPR associated protein 9 (Cas9) nuclease can be precisely guided by a single guide RNA (sgRNA) to a target a locus in the genome, upon which the nuclease will generate a double stranded break (DSB). The DSB will then be repaired by the error-prone non-homologous end joining (NHEJ) pathway, leading to introduction of random insertions and/or deletion mutations (indels) at the specific target site. These indels can result in frame shift mutations, leading to loss of protein expression. In addition to NHEJ, the less efficient homology-directed repair (HDR) pathway can be leveraged to insert additional genetic sequences or generate point mutations in a gene.
Aside from the utility as a gene editing tool, the CRISPR/Cas9 system has been adapted as a transcriptional modulation tool by linking effector proteins to a nuclease-dead version of Cas9 (dCas9). This engineered variant maintains sgRNA-directed binding to specific DNA sequences but lacks the nuclease activity and therefore does not produce DSBs. CRISPR activation (CRISPRa) is a CRISPR-based transcriptional overexpression system whereby dCas9 is fused to activator domains such as VP64-p65-Rta (VPR). In contrast, CRISPR interference (CRISPRi) is a transcriptional repression system in which dCas9 is fused to a repressor domain such as Krüppel-associated box (KRAB)1 or SALL1-SDS3.2 Thus, these various CRISPR-based modalities can be harnessed to generate a wide variety of outcomes ranging from precise gene editing and protein knockout to transcriptional up- or down-regulation of target genes. Moreover, depending on the modality or delivery method, these effects range from transient/reversible to persistent/irreversible.
Knockdown vs. Knockout
With multiple tools available to perturb or enhance gene expression, researchers have access to several methods to examine biological processes and employ orthogonal methods to validate their results. Before starting an experiment, it is important to consider the benefits and limitations of each technique. Neither siRNA nor CRISPRi changes the genetic code, instead causing temporary knockdown of the gene of interest. In contrast, CRISPRko creates a permanent knockout of the gene of interest. Therefore, if changing the genetic code is undesirable and transient gene down-regulation is sufficient, siRNA or CRISPRi would be the best solution. Nevertheless, target knockdown may not produce the same phenotype as complete loss of function.
Ease of use may also play a role in choosing which technique to use. RNAi usually entails a transfection of siRNA to reduce target gene expression. If the transfection protocol of the particular cell line has been previously optimized, siRNA delivery can rapidly assess the effect of target knockdown in that cell type. In contrast, CRISPR technologies require expression of both the Cas9 nuclease as well as the sgRNA. Fortunately, many different approaches to introduce CRISPR/Cas9 in a multitude of cell types have been developed, including: plasmid transfections, mRNA and synthetic sgRNA transfections, lentiviral transductions, and ribonucleoprotein complex consisting of Cas9 protein and sgRNA delivery.
Specificity and Off-targeting
Target specificity remains an ongoing concern for both siRNA and CRISPR. Since the discovery of RNAi, it has been observed that siRNAs could silence non-target mRNAs with limited sequence complementarity.3 However, optimization of siRNA design by filtering seed regions has led to a decrease in off-target activity. Furthermore, since siRNA uses endogenous machinery to regulate translation, it could interfere with endogenous miRNA activity, resulting in phenotypes unrelated to the targeted gene. The specificity of CRISPR systems also depends on the specificity and fidelity of the Cas9 nuclease. The Cas9 nuclease requires a protospacer adjacent motif (PAM) to cleave genomic DNA. Once Cas9 recognizes the PAM, it then interrogates the 5’ flanking DNA sequence to assess sgRNA complementarity. Previous studies have shown that 8-14 nucleotides immediately upstream of the PAM are the most critical for target site cleavage.4 Off-target activity varies with sgRNA design, ranging from 0 to 150 off-target events per sgRNA. As with RNAi, careful sgRNA design can substantially reduce off-target activity while maintaining high specificity.
Applications: Large-scale Screening
Use of multiple complementary technologies has been successfully employed to improve the confidence of hits identified in pooled screen experiments. For example, inclusion of essential genes in CRISPRko libraries can be challenging from an analysis perspective because it leads to the loss of cells. Evers et al. reasoned that this type of screening would be ideal to study the differences between CRISPRko, shRNA, and CRISPRi.5 In their screen, they targeted 46 essential and 47 non-essential genes in a negative selection screen. They found that all three systems functioned as expected and that cells were depleted when essential genes were repressed. However, the shRNA screen showed more variability compared to the CRISPRko and CRISPRi screen, whereas the CRISPRi screen revealed that not all sgRNAs can reduce all transcript variants of certain genes. While CRISPRko outperformed RNAi and CRISPRi in this screen overall, a subset of sgRNAs targeting non-coding sequences underperformed compared to sgRNAs targeting exons. Thus, this study underscores the importance of sgRNA library design for efficient screening.
Other screens may benefit from the reduced knockdown efficiency with CRISPRi to understand how subtle gene modulation can impact disease. Perdersen et al. conducted a CRISPRi screen and identified 18 genes involved in silencing HIV-1 after antiretroviral therapy.6 Since this screen was performed in a modified Jurkat cell line, they also validated these hits with siRNA in primary CD4+ T cells from HIV-1 infected individuals undergoing antiretroviral therapy.
Finally, scientists have also used whole genome pooled screening to understand biological processes in specific cell types. Gene expression can vary between cell types, but gene function can also be cell type specific. For example, mutations in proteins that are broadly expressed can lead to tissue- and cell type-specific defects and disease states. Examining gene function in different cell types is therefore important to understand tissue-specific cell biology and disease mechanisms. Tian et al. performed complementary CRISPRi and CRISPRa screens and identified genes that affect survival in neurons but not stem cells or cancer cells, supporting the notion that certain genetic diseases specifically cause neurological diseases.7 Neurons are highly susceptible to oxidative stress; thus, the authors performed a subsequent round of complementary CRISPRi and CRISPRa screens with cells subjected to oxidative stress. Numerous oxidative stress response genes were identified in these screens including the gene encoding the glycoprotein precursor prosaposin (PSAP). The authors chose to validate this hit using CRISPRko and found that PSAP knockout neurons are highly sensitive to oxidative stress, triggering ferroptosis and cell death.
These examples show that after carefully designing experiments, gene interference is a powerful method to understand biological processes in a diverse set of cells. Innovative screening practices, validated to work synergistically to enhance hit detection and generate robust datasets are also available to researchers.8 In many cases, orthogonal, or independent, validation is a requisite addition to the gene editing toolbox and can ensure that consequent findings are arrived at in the most reliable and efficient manner.
References
1. Gilbert LA, et al, 2013 CRISPR-mediated modular RNA-guided regulation of transcription in eukaryotes. https://pubmed.ncbi.nlm.nih.gov/23849981/
2. Mills C, et al, 2022 A Novel CRISPR Interference Effector Enabling Functional Gene Characterization with Synthetic Guide RNAs. https://pubmed.ncbi.nlm.nih.gov/36257604/
3. Boettcher M and McManus MT Choosing the Right Tool for the Job; RNAi, TALEN or CRISPR https://www.ncbi.nlm.nih.gov/pmc/articles/PMC4441801/
4. Hsu PD, et al, 2013 DNA targeting specificity of RNA-guided Cas9 nucleases https://pubmed.ncbi.nlm.nih.gov/23873081/
5. Evers B, et al, 2016 CRISPR knockout screening outperforms shRNA and CRISPRi in identifying essential genes https://pubmed.ncbi.nlm.nih.gov/27111720/
6. Pedersen SF, et al, 2022 Inhibition of a Chromatin and Transcription Modulator, SLTM, Increases HIV-1 Reactivation Identified by a CRISPR Inhibition Screen https://pubmed.ncbi.nlm.nih.gov/35730977/
7. Tian R, et al, 2021, Genome-wide CRISRPi/a screens in human neurons link lysosomal failure to ferroptosis. https://pubmed.ncbi.nlm.nih.gov/34031600/
8. “RNAi and CRISPRi: On-target knockdown leads to high-confidence datasets”, Horizon Discovery (2022). https://horizondiscovery.com/en/blog/2022/rnai-and-crispri