Multielement analysis is used with a wide range of samples, including water, soil, meat, fruit, vegetables, dairy products, beverages, cosmetics, pharmaceuticals, semiconductor process chemicals, metals, ceramics and geological materials. These analyses can determine the levels of elements in a sample, which is vital in the environmental and pharmaceutical industries for compliance with regulations, and also of great importance in food, materials and other markets for process optimization and quality control. Most routine multielement analyses are performed using atomic absorption spectrometry (AAS), inductively coupled plasma-optical emission spectrometry (ICP-OES) and quadrupole inductively coupled plasma-mass spectrometry (ICP-QMS). The choice of technique depends on the analytical performance required with respect to detection limits, concentration range, speed and element range for the samples (Table 1 and flow chart in Figure 1).
Table 1 – Summary of analytical performance for atomic spectrometry techniques
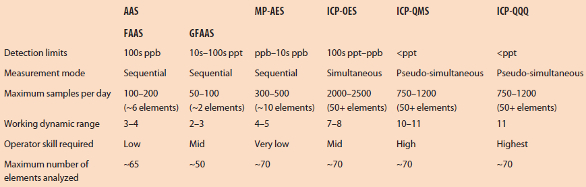
Figure 1 – Algorithm for atomic spectrometry technique selection, based on number of analytes, detection limit, number of samples measured per day and operator skills. aBetween 6 and 10 elements per sample; babove 50 elements per sample (for more details, see Table 1).
Atomic spectrometry techniques
AAS
Flame atomic absorption spectrometry (FAAS) is best suited for applications that require single-element analysis at relatively high concentrations. Although it can measure multiple elements (6–8 elements per sample is optimum), the technique tends to be slower for multielement analysis. Instrument sensitivity depends on the element, but detection limits are typically in the range of 0.1 mg/L (or parts per million, ppm). Because of the low purchase price and running costs, FAAS instruments are used in most industrial settings and have long been commercially available; as such, many online resources report on the diverse applications of FAAS.
Graphite furnace AAS (GFAAS) offers detection limits in the range of 0.1–0.01 μg/L (parts per billion, ppb). While sensitive, the technique is slow and has reduced elemental coverage compared to other atomic spectrometry techniques. GFAAS is typically used in applications that require high sensitivity and low detection limits for a small number of analytes.
ICP-OES
ICP-OES is considered a midrange, multielement technique with higher speed and a larger dynamic range than AAS. Its low detection limits (low ppb), wide linear dynamic range and simplicity of operation have contributed to its widespread use and hundreds of published applications. Recent advances in hardware and software design have addressed some of the challenges associated with ICP-OES (e.g., high instrument and infrastructure cost, and complex setup and operation), thereby streamlining workflow, reducing complexity and allowing easy data manipulation. ICP-OES has broad applications in inorganic chemistry laboratories in the environmental and food sectors and in academic settings.
ICP-MS
ICP-MS is a fast multielement technique, with the broadest element coverage, widest dynamic range and best detection limits (<ppt, parts per trillion) of any platform. Recent developments have addressed long-standing issues such as the inability to analyze higher-matrix samples and resolve problematic spectral interferences. The technique is commonly found in inorganic chemistry laboratories across most industries.
Elemental analysis in food samples
AAS, ICP-OES and ICP-QMS are well-suited for routine analyses of food and natural products. However, newer techniques like microwave plasma-atomic emission spectrometry (MP-AES) and triple-quadrupole inductively coupled plasma-mass spectrometry (ICP-QQQ) address the challenges these methods present, handling the more demanding analytical requirements while reducing the need for advanced operator skill levels and laboratory and site service.
MP-AES
Table 2 – FAAS and MP-AES detection limits (adapted from Ref. 6)
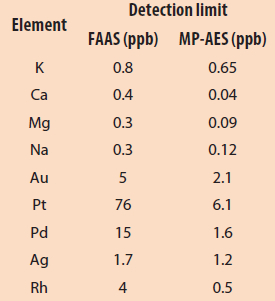
The analytical performance of MP-AES is similar to that of FAAS, but MP-AES can measure more elements more rapidly across a broader concentration range (Table 2). Its lower detection limit (0.01 ppm) results from the use of 5000 K nitrogen plasma and optics layout.
As opposed to FAAS instruments, which employ flammable gases like acetylene, MP-AES runs on nitrogen extracted directly from air, making operation safer and less expensive. MP-AES finds application in such industries as food and agriculture, environmental science and mining and in government and academic settings. Operating and purchasing costs are lower than those of high-end techniques like ICP-QMS and ICP-QQQ (see below).
Nelson et al. found MP-AES to be a cost-effective alternative to conventional ICP-MS for determining the geographic origin of Malbec wines.1 Specifically, they observed that Sr, Rb, Mg, Ca, Na and K showed excellent recovery across wide concentration ranges, which allowed these data to be used to identify the origin of wines from Argentina and California.1 Karlsson et al.2 compared the performance of MP-AES with ICP-MS and evaluated MP-AES for the analysis of principal metals (Ca, K, Mg and Na), lithogenic metals (Al, Fe and Mn) and some trace elements (As, Ba, Cd, Co, Cr, Cu, Mo, Ni, Pb, V and Zn) in sunflower samples. MP-AES successfully determined all principal and lithogenic metals, with results identical to those obtained with ICP-MS. While the concentrations of As, Co and Mo were below the detection limit for MP-AES, all other trace elements were measured successfully.2
ICP-QQQ
ICP-QQQ has lower detection limits (even for difficult elements such as S, Si and P), wider dynamic range and superior control of spectral interferences than other techniques. The disadvantages of ICP-QQQ are similar to those for conventional ICP-MS, namely, high purchase cost and the need for a skilled operator to perform method development. However, some of the operational complexity is mitigated by the fact that a method run on ICP-QQQ can be adapted across different sample types, eliminating much of the sample preparation and processing required for techniques with less tolerance for a changing matrix.
By way of contrast to ICP-QMS, ICP-QQQ uses two functioning mass spectrometers placed on either side of the collision/reaction cell. In the tandem MS configuration (MS/MS), the first quadrupole acts as a mass filter, allowing only target analytes to enter the cell and rejecting off-mass ions. This ensures effective removal of polyatomic interferences in reaction mode, delivering accurate and consistent results and providing an outstanding signal-to-noise ratio. The combination of high sensitivity, low background and effective removal of interferences allows ICP-QQQ to address applications like nanoparticle characterization and trace-level speciation.
Silica nanoparticles (SiO2 NPs) are used as food additives (E551) as well as in many industrial and consumer products, e.g., paints, polymers, toothpastes, cosmetics and detergents. Their widespread use has raised concerns about the potential effects of SiO2 NPs on biological systems and the environment. ICP-QQQ provides a new method for the determination of SiO2 NPs that is more sensitive and accurate than conventional ICP-QMS. The latter is unable to reliably measure Si isotopes at the levels and particle sizes required due to polyatomic interferences and the relatively poor signal-to-noise ratio.
ICP-QQQ in MS/MS mode was successfully adopted by Yamanaka et al. to measure SiO2 NPs.3 Time-resolved analysis of SiO2 NPs using ICP-QQQ showed an improved signal-to-noise ratio compared to ICP-QMS when measuring small (50-nm) NPs. Figure 2a shows the time-based ICP-QQQ signals measured for Si in deionized water (top chart) and a solution containing 50-nm silica NPs (bottom chart). In both plots, a section of the chart has been expanded for clarity. The “spikes” that are clearly visible above the baseline in the bottom chart represent the signals from each individual silica NP as it is atomized in the plasma. The particle peaks for the 50-nm NPs were clearly resolved and easily distinguishable from the background, demonstrating that small (50-nm) silica NPs can be detected using ICP-QQQ (Figure 2a). The high signal-to-noise and effective removal of polyatomic interferences in MS/MS mode allowed various sizes of SiO2 NP sizes to be accurately determined (Figure 2b).3
Figure 2 – a) SiO2 single nanoparticle events acquired using fast time resolution analysis (TRA) mode. Background signal was minimal (DI H2O, top panel) and allowed accurate measurements of even 50-nm (bottom panel) nanoparticles. b) Particle size distribution calculated for SiO2 NPs. (Figure modified from Ref. 3.)
ICP-QQQ was also used for the precise determination of As and Se,4 and for the measurement of the different chemical forms of As (speciation).5 This is important in many sample types, including food, environmental materials and pharmaceutical products, as the toxicity of As is highly dependent on its chemical form (inorganic As is much more toxic than the organic forms). Inorganic As has been detected in fruit juices, rice and rice products; Se, on the other hand, is an essential micronutrient, often present at insufficient levels in the diet. Precise determination of both elements is therefore critical in food samples. Rare earth elements (REEs) such as Nd, Sm and Gd can form doubly charged ions that interfere with the determination of both As and Se. ICP-QMS is not always effective at resolving the doubly charged overlaps (Figure 3), and using correction equations is not a reliable way to resolve this; rather, it can cause errors. Jackson et al. accurately determined As and Se concentrations using ICP-QQQ, as this technique enabled removal of doubly charged REE overlaps, providing a tenfold lower detection limit than conventional ICP-QMS.4
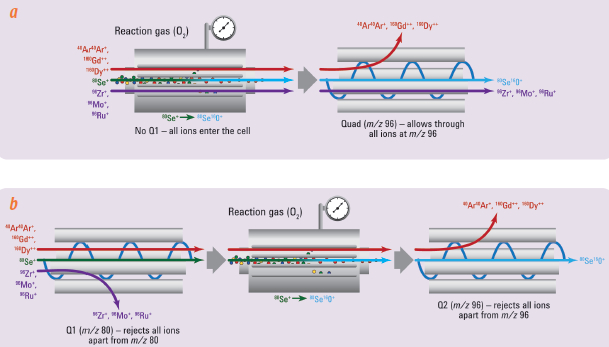
Figure 3 – Se measurement in mass-shift reaction mode: conventional ICP-QMS versus ICP-QQQ. a) In conventional ICP-QMS, Se is reacted away from some interferences (e.g., Ar
2+, Gd
++ and Dy
++) at m/z 80.
80Se is converted to
80Se
16O
+ in the cell and measured at m/z 96. Polyatomic interference still results from the presence of Zr, Mo and Ru overlapping at m/z 96. b) In ICP-QQQ, the first MS acts as a filter, allowing only ions at m/z 80 to pass to the cell, therefore excluding Zr, Mo and Ru, and reducing polyatomic interferences. (Figure modified from Ref. 3.)
Conclusion
MP-AES and ICP-QQQ extend the range of measurements that can be performed in inorganic analytical laboratories, allowing the precise determination of elements in various types of samples. The resulting analytical data are being used to support a wide range of applications in diverse fields.
References
- Nelson, J.; Hopfer, H. et al. Elemental profiling of Malbec wines under controlled conditions using microwave plasma-atomic emission spectroscopy. Am. J. Enology and Viticulture 2015, 66, 373–8.
- Karlsson, S.; Sjoberg, V. et al. Comparison of MP-AES and ICP-MS for analysis of principal and selected trace elements in nitric acid digests of sunflower (Helianthus annuus). Talanta 2015, 135, 124–32.
- Yamanaka, M.; Itagaki, T. et al. High sensitivity analysis of SiO2 nanoparticles using the Agilent 8900 ICP-QQQ in MS/MS mode. Agilent Technologies application note, 2016.
- Jackson, B.; Liba, A. et al. Advantages of reaction cell ICP-MS on doubly charged interferences for arsenic and selenium analysis in foods. J. Anal. At. Spectrom. 2014, 2015; doi:10.1039/C4JA00310A.
- Pétursdóttir, Á.H.; Friedrich, N. et al. Hydride generation ICP-MS as a simple method for determination of inorganic arsenic in rice for routine biomonitoring. Anal. Meth. 2014, 6, 5392–6.
- Pang, S. Application of a second generation microwave plasma atomic emission spectrometer (MP-AES) in the analysis of food samples; http://ll1.workcast.net/10311/0279275158671341/Documents/Pang.pdf.
Shane Elliott is director of marketing, Atomic Spectroscopy, Agilent Technologies Australia, 679 Springvale Rd., Mulgrave, Victoria 3170, Australia; e-mail: [email protected] ; www.agilent.com