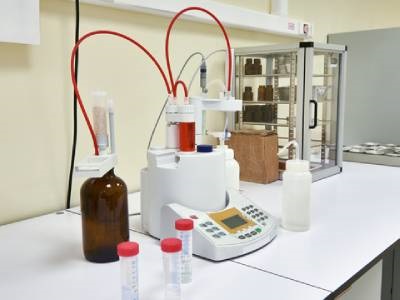
Regardless of the reaction used, all titration methods require the measurement and indication of the endpoint of the reaction, which occurs at or shortly after the equivalence point of the reaction depending on the indication method used. While the equivalence point is the point at which there is a chemically equivalent amount of the titrant and analyte in the solution, the endpoint is the time at which the indicator marks the end of the reaction through a color change or other signal. The measurement will be more precise the closer the endpoint is to the equivalence point.
The three most common methods used for reading the endpoint of a titration reaction are color-change (manual), potentiometric and thermometric. This article explains these three main methods, how they work and how to decide which method may be a good fit for your application.
Manual Titration (Color Change)
When titrations are performed manually, typically, a color changing indicator is used to signal the endpoint of the reaction, and this change is observed with the naked eye. The type of indicator used depends on the reaction type and can also depend on the analyte and titrant involved in the reaction. For example, phenolphthalein is often used as an indicator for acid-base titrations when the titrant is a strong base because phenolphthalein changes from colorless to pink at a pH of around 8.3.1 Methyl orange, which changes from yellow to red between pH 3.1 and 4.4, is frequently used as an indicator for reactions between a strong acid titrant and strong base analyte.
Indicators for redox titration reactions are more specific to the oxidizing and reducing agents involved. Some redox indicators change color after reacting with the titrant, such as when starch reacts with iodine and turns blue at the end point of iodimetry. Other redox indicators change color in reaction to changes in electrode potential, in which case the indicator must be selected based on the expected potential of the solution at the equivalence point of the redox reaction. The equivalence point potential can be estimated using the Nernst equation and the standard reduction potentials of the half-cells of the reactants, and will fall approximately in between these two half-cell potential values. For example, the half-reaction potential of Fe3+/Fe2+ is 0.77 V and the half-reaction potential of Ce4+/Ce3+ is 1.44 V, so ferroin, which changes color from red to blue when oxidized at a potential of 1.06 V, is commonly used as an indicator when Fe2+ is titrated with Ce4+.
Some redox titrants can also serve as their own indicators, such as potassium permanganate (KMnO4). During titration, MnO-4 from the purple or pink KMnO4 solution is colorless when reduced to manganese(II) (Mn2+) by the titrand. When there is excess MnO-4 in the sample solution, the solution turns pink, marking the end point of the reaction.
For precipitation reactions, indicators may change color by reacting with the titrant or the precipitate. For example, in the Mohr method, where silver ions (Ag+) from silver nitrate (AgNO3) react with chloride ions (Cl-) to form a silver chloride (AgCl) precipitate, potassium chromate (K2CrO4) is added and reacts with excess silver ions to form a reddish-brown silver chromate (Ag2CrO4) precipitate, signaling the end of the reaction. In the Fajans method, which also uses AgNO3 to titrate chloride, a dichlorofluorescein dye is used as the indicator, which turns from green to pink when adsorbed onto the surface of the AgCl precipitate. This adsorption and color change can only occur once all the chloride ions have reacted, causing excess Ag+ to positively charge the precipitate’s surface and attract the anionic dye.
In complexometric titration, indicators are usually ionochromic dyes that form a weak complex with the metal ions being analyzed and differ in color depending whether they are in a complex or not. When a stronger complexing or chelating agent, such as EDTA, sequesters the metal ions, the dye returns to its original color and indicates a complete reaction. For example, Fast Sulphon Black F is a dye that changes from purple to green when it is no longer complexed with copper ions.
Manual titrations that rely on the experimenter to visually detect a color change are more prone to error than automated titration methods, and perceptions of color change may differ from person to person. However, manual titrations using color-changing indicators are simpler and less expensive to perform than automated methods, at least in terms of upfront equipment costs. The precision and accuracy of manual titration methods can be greatly improved with both proper training and proper indicator selection. Using indicators with a fast and clear color change, with suitable chemistry for the titration reaction being indicated, can reduce the possibility of error due to subjective color perception or late/early indication. Additionally, one should ensure that the indicator will not interfere with the reaction between the titrant and the analyte.
Potentiometric Titration
In potentiometric titration, the potential difference between two electrodes is used to determine the equivalence point of the titration reaction. An indicator electrode, which is immersed in the sample solution, is connected to a reference electrode by a salt bridge containing an inert electrolyte such as potassium chloride (KCl), forming an electrochemical cell.3 The potential of the reference electrode is constant while the potential of the indicator electrode changes depending on the ions present in the sample solution. The potential of the cell is monitored as the titrant is added, and plotted as a function of the volume added. Because the potential is dependent on the concentrations of the analyte and titrant in the solution, the plot can be used to determine the equivalence point, found at the steepest section of the potentiometric titration curve.
Potentiometric titration can be used for virtually any type of titration reaction (acid-base, redox, precipitation and complexometric), but suitable electrode types must be selected based on type of reaction and reactants involved. For example, pH electrodes (ex. glass indicator electrode and silver/silver chloride reference electrode) are ideal for acid-base titration reactions. Platinum indicator electrodes with calomel or silver/silver chloride (Ag/AgCl) reference electrodes are typically used for redox reactions, while silver indicating electrodes are often paired with mercurous sulfate reference electrodes in precipitation titrations.4 Complexometric titrations require specific electrodes that are selective for the ion being analyzed. Some ion-selective electrodes (ISEs) are commonly available, such as ISEs selective to copper and cadmium, while some analytes may require electrodes to be specially prepared to ensure selectivity. For complexometric titrations using EDTA as a titrant, another option is to prepare mercury-coated electrodes by amalgamating silver or gold electrodes.
Potentiometric titration is more precise than manual titrations using color-changing indicators, as it is less subjective and the equivalence point can be pinpointed with greater accuracy using the potentiometric titration curve. Potentiometric titration can also enable greater versatility, as the same electrodes can be used for different titrations in many cases, whereas indicator-based titration is more dependent on the chemistry of the indicator used. Potentiometric titration is used in many automated titrators, reducing human error, variability and labor and generally increasing speed and throughput via continuous automatic operation. The upfront costs of an automated potentiometric titrator are much higher than that of manual titration, which requires little more than a burette, flask and the necessary reagents, but may be worth it for labs with a high testing volume or applications that require more precise measurements. Additionally, an automated titrator may save costs over time due to the reduction in manual labor. Removing the need to purchase multiple different indicators may also be a benefit, but the costs, maintenance and availability of the required electrodes should also be considered.
Thermometric Titration
Thermometric titration uses temperature changes, rather than color or potential changes, to determine the equivalence point of a titration reaction. This method is based on the enthalpy changes that occur during a chemical reaction. In exothermic titration reactions, energy released by the reaction causes the temperature of the solution to increase as the titrant reacts with the analyte and decrease when the reaction is complete.5 In endothermic reactions, energy is absorbed and the temperature decreases, then stabilizes or increases again at the equivalence point of the reaction. Because the temperature changes for many titration reactions are extremely small, high-resolution temperature probes are required for accurate thermometric titration measurements. Temperature as a function of titrant volume added can be plotted as the reaction is monitored, and the equivalence point can be identified as the point when the temperature change stops or reverses direction.
One of the main benefits of thermometric titration is the fact that a single temperature probe can be used for any reaction type, given that the sensitivity of the probe is sufficient to detect the temperature changes. Thermometric titration is also easily automated, avoiding the problems associated with manual titration. Thermometric titration could also be applied in situations where suitable indicators or electrodes are not available for the specific analyte being studied. Limitations of thermometric titration include the costs of a highly-sensitive temperature probe or automated system, as well as the potential interference of external temperature fluctuations on the experiment.
References
- Harvey, D. 9.4: Redox Titrations. In Analytical Chemistry 2.1.; LibreTexts Chemistry; Open Education Resource LibreTexts Project, 2021. https://chem.libretexts.org/Bookshelves/Analytical_Chemistry/Analytical_Chemistry_2.1_(Harvey)/09%3A_Titrimetric_Methods/9.04%3A_Redox_Titrations
- Precipitation Titration. In General Lab Techniques; LibreTexts Chemistry; Open Education Resource LibreTexts Project, 2021. https://chem.libretexts.org/Ancillary_Materials/Demos_Techniques_and_Experiments/General_Lab_Techniques/Titration/Precipitation_Titration
- Harvey, D. 11.2: Potentiometric Methods. In Northeastern University; LibreTexts Chemistry; Open Education Resource LibreTexts Proect, 2019. https://chem.libretexts.org/Courses/Northeastern_University/11%3A_Electrochemical_Methods/11.2%3A_Potentiometric_Methods
- "Titration Theory and Practice," Hach (2014). https://www.hach.com/asset-get.download.jsa?id=24124425653
- "Themometric titration — the missing piece of the puzzle," Article by Lucia Meier, Metrohm (2020). https://www.metrohm.com/en_us/discover/blog/20-21/thermometric-titration---the-missing-piece-of-the-puzzle.html