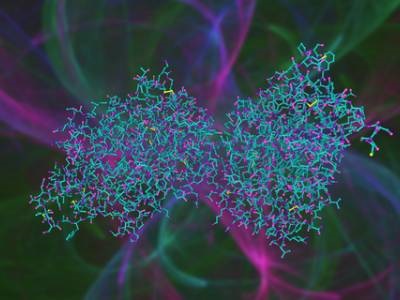
by Pamela James, Ph.D., Vice President, Product, Vector Laboratories
Realizing the dream of truly personalized medicine is a dominant goal in the biomedical research landscape. However, reaching this milestone is not only about advancing therapeutics for rare and complex diseases—a deeper understanding of disease mechanisms and improvements in our capacity to identify them will be necessary steps in the mission. Biomarker discovery is a fundamental arm of progress in personalized medicine that has been revolutionized by advances in exploring genomic, proteomic, and metabolomic markers of health and disease. One promising sector remains largely unexplored: glycomic biomarkers, which encompass the glycans and patterns of glycosylation present in the body.
Glycans are complex, branched chains of polysaccharides and oligosaccharides that join to proteins and lipids in a process called glycosylation. Their presence throughout cells and tissues and their role in maintaining normal biological functions, from facilitating vital cell-to-cell interactions to guiding protein folding, make glycans an ideal potential class of biomarkers for numerous diseases. Research in detecting and characterizing dysregulated glycosylation and its impact on downstream processes will be a key contribution to the development of new diagnostics and therapeutics. Although recent technical developments have expanded our capabilities for studying the field, glycobiology remains in its infancy relative to other sectors. It has long been considered a highly specialized field of research, but several decades of slow but steady growth have expanded the footprint of glycobiology in the broader research landscape.
In this article, I’ll provide a basic overview of the fundamentals of glycobiology and its role in health and disease. Additionally, I will review the characteristics that make glycans and glycosylation strong potential candidates for biomarker discovery as well as the latest advances in research methods powering new applications for glycobiology.
Basics of Glycobiology
Glycosylation is a common post-translational modification (PTM) that results in the covalent attachment of glycans to specific proteins, lipids, or other molecules. The process is ubiquitous throughout cells and tissues, with an estimated 50-70% of all proteins bearing glycans and approximately 2% of human genes coding for proteins related to glycosylation in some capacity.1 As with phosphorylation, another very common PTM, glycosylation can act as a real-time modulator of cellular signaling and protein function. These PTMs allow cells to respond to environmental cues and fine-tune cellular processes across biological systems and are thus heavily implicated in disease etiology and progression.2
Glycans come in many forms, but the two most prevalent types of glycosylation are N-linked and O-linked. These classes of glycosylation differ by their binding sites on biological molecules. While N-linked glycans join via nitrogen atoms located within asparagine residues on proteins, O-linked glycans attach to oxygen atoms of hydroxyl groups located on serine or threonine residues. Interestingly, the serine and threonine residues binding O-linked glycans are also potential phosphorylation sites, further demonstrating the close relationship of these PTMs in biological regulation.3
Glycosylation is Diverse and Dynamic
The glycoforms resulting from N- and O-linked glycosylation are extremely diverse. For example, a single glycoprotein can contain numerous potential N-linked glycosylation sites, which may or may not be occupied, and O-linked glycans may attach to any serine or threonine residue. This diversity is also a result of the dynamic influences on glycosylation and the nearly endless combinations of sugar building blocks, attached chemical moieties, and bonds that join them. Unlike proteins, glycan structure is not coded by any set template but is instead regulated by the local milieu of enzymes. Because of this, the population of glycans present in any given site is extremely heterogenous and constantly changing.4 The distinct characteristics of glycans can have a profound effect on the structure and function of proteins, in turn impacting any downstream processes they regulate.
One example of the complex regulatory role of glycosylation is in immune function, where glycans serve as a fundamental cellular marker of “self” vs. “other.” Additionally, N-linked glycans are vital to guiding proper protein folding. Chaperone proteins in the endoplasmic reticulum bind to N-linked glycans on the surface of new proteins, influencing their folding. Certain patterns of glycan placement on these proteins can encourage or inhibit specific folding arrangements, which can be vital in determining the proteins’ ultimate function. Glycosylation is also an important modulator of cell signaling and regulation. For example, glycan placement can regulate the function of growth factor receptors on the cell surface and target proteins for delivery to the lysosome for degradation.5
Small Shifts in Glycosylation, Major Implications for Disease
Even minor changes to glycosylation or glycan structure can significantly dysregulate biological processes, impacting health and disease. Normal glycosylation depends on the synthesis of carbohydrate components, the activity of various enzymes, and the perfectly coordinated function of organelles, including the endoplasmic reticulum and Golgi.6 Because glycosylation is such a complex and dynamic process, glycan changes can indicate perturbations in upstream processes or serve as a signal of downstream effects on other functions. The multifaceted role of glycans within these tightly interwoven biological factors and processes makes them a promising potential class of biomarkers or therapeutic targets.
Diseases directly impacting glycan synthesis and modification pathways, known as congenital disorders of glycosylation (CGDs), are their own category of rare genetic diseases. CGDs encompass a wide variety of multisystem symptoms and disorders across a spectrum of severity, though neurological issues are present in most cases. The advent of next-generation sequencing approaches has made diagnosis of the more than 150 distinct CGDs significantly more accessible to patients and clinicians.7
Aberrant glycosylation is a component of many other disease mechanisms not directly related to abnormal synthesis and modification of glycans. Every immune cell is coated with an assortment of glycoproteins, glycolipids, and glycan-binding proteins called lectins. Dysregulation of normal glycosylation of any of these entities can have deleterious consequences on the control of both innate and adaptive immune cell activity.8 For example, the glycosylation of immunoglobulins (Ig) is a key modulator of inflammation, such that aberrant glycosylation can act as a “switch” in the development of chronic inflammatory and autoimmune disorders.9
Glycosylation is also known to influence the development and progression of cancer, regulating the interaction of immune and tumor cells as well as tumor cell signaling, migration, and proliferation.10
Leveraging Glycobiology to Discover Novel Biomarkers
Existing research on glycosylation in health and disease has already yielded useful biomarkers, and the landscape will continue to expand as our knowledge and research capabilities expand. Glycan profiles have shown to be a valuable tool in the diagnosis of conditions including lupus, inflammatory bowel disease, rheumatoid arthritis, and more.11 Progress has also been made in identifying glycan- and glycoprotein-based biomarkers for the detection and characterization of cancer. Antibodies against tumor-specific glycans, abnormally large N-linked glycans, and aberrant expression of glycosyltransferases have already been leveraged as biomarkers for certain types of cancer.12,13 Glycan-associated biomarkers have also been identified for cardiovascular diseases, Parkinson’s disease, and other conditions.14,15
Methodological Advances in Glycobiology Research
While glycobiology is slowly approaching a more mainstream status in biomedical science, much of the glycome remains a mystery to researchers. Normal glycosylation is related to countless upstream and downstream processes in every cell and tissue type, so identifying the source or consequences of disrupted glycosylation can be challenging. The production and modification of glycans depend on the coordinated function of multiple organelles and enzymes, as described previously. However, the proteins and lipids that accept glycans are also influenced by the same range of factors, meaning that countless variables are at play in the study of glycosylation.
Fortunately, new methods have advanced our capacity to unravel the complexities of glycobiology in health and disease, allowing researchers to identify new biomarkers more efficiently. Mass spectrometry (MS) has been a vital and highly sensitive tool in screening for changes in glycan structure. Recent developments in MS technology, such as liquid chromatography MS (LC-MS) and matrix-assisted laser desorption/ionization MS (MALDI-MS), have enabled glycan-based biomarker discovery in plasma, serum, and tissue samples. These updated approaches have helped researchers characterize aberrant glycosylation in pancreatic cancer, hepatocellular carcinoma, breast cancer, and other conditions.16
Leveraging whole-glycome data in biomarker discovery may be a more useful strategy than the identification of single indicators of disease. This approach has been facilitated by innovations in bioinformatics and multi-omics techniques, such as the development SUrface-protein Glycan And RNA-seq (SUGAR-seq). By combining the power of single-cell RNA sequencing with post-translational modification data, SUGAR-Seq enabled more detailed insights into the functional and epigenetic state of individual cells.17 Additionally, new technology has increased the viability of glycan-based diagnostics by increasing the scalability and efficiency of analysis methods. Lectin-based biosensor microarrays and lectin/antibody proximity ligation analyses are two such examples, opening the door to simple, portable, and sensitive biomarker detection methods that could be employed on a point-of-care basis.18,19
Paving the Way for Glycan Biomarker Discovery
Intimately involved in the regulation of countless biological functions and present throughout cells and tissues, glycans hold massive potential for biomarker discovery. Aberrant glycosylation has already been implicated in many diseases, and ongoing research will elucidate other glycomic insights in disease etiology, progression, and potential therapeutic options. While the complexity of this field has made its growth slow and steady, recent technological advances in studying glycosylation have powered incredible discoveries. With continued dedication to researching the secrets of the complex sugars influencing nearly every biological process, we can move closer to leveraging their full potential in science and medicine.
References
- Apweiler, Rolf, Henning Hermjakob, and Nathan Sharon. "On the frequency of protein glycosylation, as deduced from analysis of the SWISS-PROT database." Biochimica et Biophysica Acta (BBA)-General Subjects 1473.1 (1999): 4-8.
- Ardito, Fatima, et al. "The crucial role of protein phosphorylation in cell signaling and its use as targeted therapy." International journal of molecular medicine 40,2 (2017): 271-280.
- Bektas, Meryem, and David S Rubenstein. “The role of intracellular protein O-glycosylation in cell adhesion and disease.” Journal of biomedical research vol. 25,4 (2011): 227-36.
- An, Hyun Joo et al. “Determination of glycosylation sites and site-specific heterogeneity in glycoproteins.” Current opinion in chemical biology vol. 13,4 (2009): 421-6.
- Varki, Ajit. “Biological roles of glycans.” Glycobiology vol. 27,1 (2017): 3-49.
- Sobral, Daniel et al. “Concerted Regulation of Glycosylation Factors Sustains Tissue Identity and Function.” Biomedicines vol. 10,8 (2022) 1805.
- Lipiński, Patryk, and Anna Tylki-Szymańska. “Congenital Disorders of Glycosylation: What Clinicians Need to Know?.” Frontiers in pediatrics vol. 9 (2021): 715151.
- Reily, Colin, et al. "Glycosylation in Health and Disease." Nature reviews. Nephrology, vol. 15, 6 (2019): 346.
- Zhou, Xing et al. “Antibody glycosylation in autoimmune diseases.” Autoimmunity reviews vol. 20,5 (2021): 102804.
- Pinho, S., Reis, C. Glycosylation in cancer: mechanisms and clinical implications. Nat Rev Cancer vol. 15 (2015): 540-555.
- Ząbczyńska, Marta et al. “Glycosylation in Autoimmune Diseases.” Advances in experimental medicine and biology vol. 1325 (2021): 205-218.
- Andergassen, Ulrich, et al. "Glycosyltransferases as markers for early tumorigenesis." BioMed research international 2015 (2015).
- Tikhonov, Aleksei, Smoldovskaya, Olga, Feyzkhanova, Guzel, Kushlinskii, Nikolay and Rubina, Alla. "Glycan-specific antibodies as potential cancer biomarkers: a focus on microarray applications" Clinical Chemistry and Laboratory Medicine (CCLM), vol. 58, 10 (2020): 1611-1622.
- Scott, David W et al. “Heterogenic endothelial responses to inflammation: role for differential N-glycosylation and vascular bed of origin.” Journal of the American Heart Association vol. 2,4 (2013): e000263.
- Váradi, Csaba, et al. “Serum N-Glycosylation in Parkinson’s Disease: A Novel Approach for Potential Alterations.” Molecules, vol. 24, no. 12, June 2019, p. 2220.
- Wang, Mengmeng, Zhu, Jianhui, Lubman, David M. and Gao, Chunfang. "Aberrant glycosylation and cancer biomarker discovery: a promising and thorny journey" Clinical Chemistry and Laboratory Medicine (CCLM), vol. 57,4, 2019, pp. 407-416.
- Kearney, C.J. et al. “SUGAR-seq enables simultaneous detection of glycans, epitopes, and the transcriptome in single cells” Science advances, vol. 7,8, 2021.
- Silva, M Luísa S. “Lectin biosensors in cancer glycan biomarker detection.” Advances in clinical chemistry vol. 93, 2019, 1-61.
- Robinson, Peter V., et al. “Glyco-Seek: Ultrasensitive Detection of Protein-Specific Glycosylation by Proximity Ligation Polymerase Chain Reaction.” Journal of the American Chemical Society, vol. 138, no. 34, 2016, pp. 10722–10725.
About the Author: Pamela James, Ph.D., serves as Vice President, Product, for Vector Laboratories where she oversees research and development and leads quality assurance, product, and program management. James has spent over a decade and a half with Vector Laboratories in multiple scientific and director roles. James led the introduction of several impactful products from Vector Laboratories and is focused on Vector’s mission to bring glycobiology tools to the broader scientific community. She earned a Ph.D. in Immunology from UMass Chan Medical School and a B.S. in Biochemistry from California Polytechnic State University-San Luis Obispo.