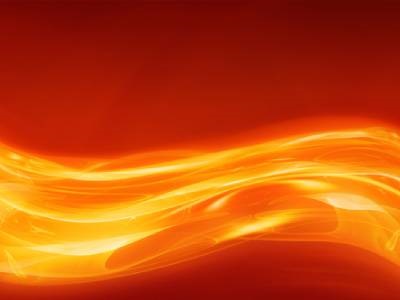
In the field of thermal analysis, differential scanning calorimetry (DSC) and differential thermal analysis (DTA) are two similar but distinct techniques used to characterize samples by measuring their response to heat in comparison with an inert reference. This differential response can provide a range of information on a material’s physical properties, including melting point, glass transition temperature and crystallization temperature. Additionally, DSC and DTA can be used for quality control purposes, for example, by detecting a deviation from a material’s expected melting point due to degradation. DSC and DTA differ in a number of ways, including in instrumentation and the level of information that can be derived from the data each technique provides. This article explains some of the similarities and differences between these thermoanalytical techniques and provides some guidance on which technique may be best for your application.
What is Differential Scanning Calorimetry?
Differential scanning calorimetry (DSC) measures the heat flow in and out of a sample in comparison to a reference. A DSC instrument will detect changes in heat flow during events such as phase transition or chemical reaction. How heat flow is determined differs between the two most common types of DSC: heat flux and power-compensation.
A heat flux DSC instrument measures the temperature difference between a sample and reference connected by a low-resistance heat flow path and heated together in the same furnace under a controlled temperature program.1 The flow path is formed by a thermoelectric material, such as disk of copper-nickel alloy, through which heat is transferred to and from the sample and reference. By measuring the differences in temperatures between the sample and reference over time, analysts can ultimately calculate the differential heat flow and identify endothermic and exothermic processes in the sample.
A power-compensation DSC method, by contrast, involves the use of two separate furnaces, allowing the sample and the reference to be heated independently. The temperatures of both materials are constantly monitored as they are heated, and power to each furnace is increased or decreased so that the temperatures of the sample and reference are equivalent throughout the experiment. Heat flow can be easily derived from this data because it is directly proportional to the power differential between the furnaces.
Both heat flux and power-compensation can be used to produce a thermogram plotting the heat flow differential against system temperature or time. Upward peaks in the thermogram represent endothermic events (ex. melting), while downward peaks represent exothermic events (ex. crystallization), or vice versa, depending on the system or software settings used. A DSC thermogram can be used not only to determine temperatures of melting, glass transition, crystallization and more, but also to precisely quantify the enthalpies of these transitions using the calorimetric constant of the instrument and the integrated area of a peak.
What is Differential Thermal Analysis?
Differential thermal analysis (DTA) also involves the measurement of both a sample and a reference under a controlled temperature program, but in this case, the temperature differential between the two materials is the focus rather than the heat flow. In a DTA instrument, the sample and reference are placed in the same furnace, but unlike in heat flux DSC, a thermoelectric element is not used to create a heat flow path between the two. Heat flow to both the sample and reference remains constant throughout the experiment, and the temperatures of both are measured and compared to produce a thermogram.
A DTA thermogram plots the temperature differential between the sample and reference against system temperature or time. Similarly to DSC thermograms, DTA curves can help identify endothermic and exothermic events based on the types of peaks present. It is very common for DTA to be performed using a simultaneous thermal analyzer (STA) as opposed to a standalone DTA system; STAs often integrate thermogravimetric analysis (TGA) with DTA, allowing the mass of the sample to also be measured throughout the experiment.
Applications of DSC vs. DTA
DSC and DTA are used in many of the same industries, including polymer, ceramic, cement, food and pharmaceutical manufacturing. Both techniques also study similar properties and processes, such as melting point, crystallization, glass transition, curing conditions, purity and degradation. However, there are certain benefits and drawbacks of each technique to consider when selecting a method for your analysis.
One of the main differences is that DSC can be used to obtain greater quantitative detail about physical processes or reactions than DTA, including much more precise and accurate measurements of enthalpy. For example, as previously mentioned, the enthalpy of a transition or reaction, such as the enthalpy of fusion, heat of reaction, or even the enthalpy of protein unfolding, can be calculated from the calorimetric constant of the instrument and the integrated area of a peak. These additional metrics can be useful in many applications, for example, in estimating the crystallinity of a ceramic or polymer material; in this case, the heat of fusion of the sample can be compared to established reference values for 100% crystalline counterparts, data which is available for many materials.
Another area in which DSC excels is in the detection of weak or subtle transitions or reactions; for example, a glass transition appears as a baseline shift, rather than a peak, on a thermogram, which can be difficult to identify in materials such as high density polyethylene (HDPE) and other highly crystalline materials, in which glass transition may be very weak. DSC is more sensitive than DTA, making it easier to interpret subtler thermogram features. Identification of weak or overlapping reactions or transitions can be further enhanced by the use of specialized DSC techniques like temperature-modulated DSC, in which a linear temperature program is transformed to include sinusoidal oscillations. This allows both reversing and non-reversing heat flow to be calculated and plotted separately; because glass transition is related to reversing heat flow, it can easily be distinguished from overlapping processes like enthalpy relaxation when temperature-modulated DSC is used. Many modern DSC instruments and software programs include temperature-modulated DSC capabilities.
Sample Considerations for DSC vs. DTA
Smaller sample sizes are typically used for DSC, which can have both benefits and drawbacks. Due to the higher sensitivity of DSC, samples as small as 3 mg can be used for applications such as purity testing. Using a smaller sample reduces the likelihood of a temperature gradient across the sample, and can also allow for a faster analysis, especially in power-compensation DSC, where the sample is contained within its own small furnace. However, using a smaller sample amplifies the effects of errors such as decomposition during measurement. Small sample size can also create a challenge with reproducibility when the material being tested is highly heterogeneous, making a smaller sample less likely to be representative of bulk material. As many DTA and STA instruments can accommodate higher sample sizes, this aspect should be considered when testing, for example, samples with a low decomposition temperature.
While temperature ranges vary from instrument to instrument, the DTA method is typically more amenable to higher temperatures (above 800°C) than DSC. For many applications, such as the study of polymers or organic materials, temperatures this high are not required; however, for certain applications, such as thermal analysis of metal alloys, temperatures in excess of 1000°C may need to be employed to study the desired transitions or reactions. Considering the need, or lack thereof, for the additional details afforded by DSC analysis, one should consider whether a DSC instrument with an extended temperature range is necessary when a standard DTA or STA instrument would otherwise be suitable.
Conclusion
DSC and DTA are both well-established and invaluable techniques in the thermal testing of various materials. DTA sets the foundation for DSC, while the latter expanded the scope of what can be achieved through differential thermal measurements. Still, DTA remains a relevant and robust analysis method, especially when coupled with complementary techniques such as TGA. When selecting a method, one should consider the information they hope to obtain, the types of samples they will be testing and the costs and specifications of individual instruments. For applications that only require limited phase transition information, such as melting or crystallization temperatures, DTA can be a straightforward solution for routine testing. On the other hand, DSC can take thermal testing to the next level with more accurate quantification of enthalpy changes, sensitive detection of weak thermal processes, and overall more comprehensive detail about the physical properties and thermodynamics of materials.
References
1. “What Do Polymers, Pharmaceuticals, Foods, Metals, and Ceramics Have in Common? Differential Scanning Calorimetry,” Article by Kadine Mohomed, Labcompare (2018). https://www.labcompare.com/10-Featured-Articles/352511-What-Do-Polymers-Pharmaceuticals-Foods-Metals-and-Ceramics-Have-in-Common-Differential-Scanning-Calorimetry/