Featured Article
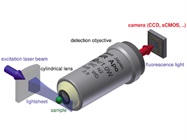
Super-resolution microscopy encompasses several new techniques for direct spatiotemporal imaging of structural features much smaller than the diffraction limit of light (about 100 nm). Atomic electron tomography (AET) is probably the leading example, with a minimum feature size of about 0.5 Å.1 AET works well on crystals and solids, but super-resolution microscopy can be used in life sciences to characterize spatiotemporal structure and function. The endoplasmic reticulum (ER) is a thoughtful target, since this organelle is essential to cell function, and previous studies have produced confounding results.
The ER was first recognized in 1945 as an artifact appearing in 2-D gel electrophoresis. Fibroblasts were so tightly bound to the ER membrane that they did not migrate normally. While the ER has been studied, most structure/function investigations have lacked spatial resolution. In addition, most sample preps kill the cells, so the temporal resolution studies are not possible.
The ER is one of the organelles to which proteins migrate after being synthesized in the ribosome. One function of the ER is the addition of post-translational modifications (PTMs) to proteins. PTMs are essential for protein function. The ER is also involved in calcium ion signaling and regulation, mitochondrial division and lipid synthesis.
A dozen scientists collaborated to study the ER using five super-resolution techniques.2 None of the techniques on its own can provide a complete description, but combined, they show a dynamic new structure that differs from prior models. According to the researchers,
Conventional optical imaging technologies had led to misidentification of these structures as sheets because of the dense clustering of tubular junctions and a previously uncharacterized rapid form of ER motion. The existence of ER matrices explains the previous confounding evidence that had indicated the occurrence of ER “sheet” proliferation … .2
Figure 1 – Schematic of laser light sheet imaging. The laser is focused on a cylindrical planar lens that produces a thin planar light beam. This illuminates the sample, which is imaged in 2-D. The narrow light source facilitates high-resolution imaging at different layers in the z axis. The composite is a 3-D spatial image. Image reproduced from Wikipedia.The new picture of the ER is a heterogeneous 3-D matrix permeated with small tubes. In live cells, these tubules move up to 70-nm range; the standard deviation is ±50 nm with a frequency of 4 Hz. Significant variations in z topography were also observed, indicating that the ER is not a smooth membrane. Indeed, lattice light sheet-point accumulation for imaging in nanoscale topography (LLS-PAINT) (Figure 1) and focused ion beam-scanning electron microscope (FIB-SEM) imaging showed that the structure is quite heterogeneous, with regions of low and high porosity. However, ER folding and stacking can be seen near the cell’s nucleus. The heterogeneity may be related to the varied functions of the ER.
The scientists used the following techniques2 to study the ER.
Structured illumination microscopy (SIM)
SIM provided 3-D images of fixed cells via an ELYRA S1 (SR-SIM) super-resolution microscope from Carl Zeiss Microimaging (Thornwood, N.Y.).
Grazing incidence-structured illumination microscopy (GI-SIM)
GI-SIM showed that the previously identified sheet structure was actually “riddled with spaces.”2 The technique provided temporal images of movement of the peripheral region of the ER. This movement is complex and perturbed in amplitude and period by various drugs. For GI-SIM, a SIM platform was fitted with total internal reflection fluorescence (TIRF) optics. The incident angle on the beam was adjusted to improve penetration depth of field to about 700 nm. Under these conditions, the incident beam forms a light sheet parallel to the coverslip surface. Careful tuning reduced the background of the TIRF image while focusing on the ER image. Time-lapse photography with bleach correction generated the images using standard TIRF-SIM software.
Confocal microscopy
Images of live cells were taken using an Eclipse Ti-E inverted microscope (Nikon Instruments, Melville, N.Y.) with a Yokogawa (Sugarland, Texas) spinning disk scan head. For fixed cells, images at various excitation lines were recorded on a Zeiss LSM 780 laser scanning confocal microscope.
Airyscan confocal imaging3
Airyscan confocal imaging was performed with a Zeiss LSM 880 fitted with an Airyscan module. The improved light throughput of the Airyscan enables higher speed and resolution of fluorescent labels.
LLS-PAINT
LLS-PAINT was achieved with a custom-built microscope. After collecting data for 14 days, the final image was reconstructed from 548,792 and 627 molecular localizations with a median precision of 7.2 nm laterally and 41.0 nm axially. The experimental and supplemental information sections in Ref. 2 also describe various image processing and filter programs.
Conclusion
While recent findings have taught us a great deal more about the structure of the ER, how it performs its tasks remains a mystery. For example, what happens in the tubules to facilitate post-translational modification? What part of the structure is responsible for lipid synthesis? On the molecular level, how is calcium regulated? With 4-D images, perhaps we can tease apart the how and where of the ER’s many functions.
References
- https://www.americanlaboratory.com/Blog/330375-Atomic-Electron-Tomography-Provides-3-D-Atomic-Structures-of-Solids/
- Nixon-Abel, J.; Obara, C.J. et al. Increased spatiotemporal resolution reveals highly dynamic dense tubular matrices in the peripheral ER. Science Oct 2016, 354(6311); doi: 10.1126/science.aaf3928.
- http://www.zeiss.com/microscopy/us/products/confocal-microscopes/lsm-880-with-airyscan-.html
Robert L. Stevenson, Ph.D., is Editor Emeritus, American Laboratory/Labcompare; e-mail: [email protected]