Featured Article
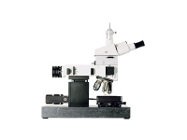
Please check out our Raman Spectroscopy section for more information or to find manufacturers that sell these products.
How do you characterize a material without touching it? That's a problem Karen Trentelman faces on a regular basis. A senior scientist at the Getty Conservation Institute in Los Angeles, Trentelman probes priceless works of art to uncover how they were made, with what materials, and whether and how those materials are breaking down. Needless to say, sampling is out of the question; hers is a case of "look, but don't touch."
"We don't experiment on the art," she says. Fortunately, she doesn't have to, thanks to Raman spectroscopy.
Raman spectroscopy uses laser energy to molecularly "fingerprint" materials nondestructively and without contact. As a result, the technique is finding use in fields as diverse as homeland security, biomedicine, pharmaceutical manufacturing, and materials science.
"As an analytical tool Raman is very powerful and very broadly applicable. It can be applied in any number of fields," says Ed Gooding, spectroscopy product manager at Princeton Instruments.
Like its analytical cousin, infrared spectroscopy, Raman detects the vibrational signature of chemical bonds, explains Andrew Whitley, vice president of sales and Raman spectroscopy at HORIBA Jobin Yvon.
In IR spectroscopy, polychromatic infrared light is directed at a sample, whose molecular bonds absorb that light at characteristic frequencies. Using the resulting absorbance spectrum, researchers can reconstruct the material's chemical composition.
Raman, in contrast, uses monochromatic light ranging from the UV into the NIR (typically laser light). As that light is directed at a sample, the sample's molecular bonds absorb the energy, become excited, and then release it. In most cases, the released light is of precisely the same energy (that is, wavelength) as the excitation source, and is called "Rayleigh-scattered"; occasionally, however – about once in every 10 million photons or so – the bonds absorb a small amount of energy, shifting the wavelength of the scattered light.
Like an NIR spectrum these "Raman-scattered" photons are diagnostic of particular chemical structures and thus can serve to identify them.
"You are measuring the same thing as in IR but through a different process," says Whitley.
And you can do so in a completely non-contact, non-destructive way – assuming you can see what you want to scan.
"The nice thing about Raman is you can analyze liquids, powders, or gels, and you can even do it through glass or plastic, such as a bag or cuvette," says Jorge Macho, Director of Technical Sales and Service at Ocean Optics. In other words, pharmaceuticals and liquids can be probed in their bottles, and suspicious white powders can be scanned within their plastic baggies. "You just change the focal point of the system to make sure the laser and collection points is pointing at the sample and not the container."
Comprising four main components, a probe (sampling optics), a laser, a filter, and a detector, Raman spectrometers come in four basic flavors: Raman microscopes, Raman macro-measurement spectrometers with or without fiber-optic probe heads for remote monitoring, and self-contained, handheld spectrometers. Each operates on the same physical principle, but microscope-based systems provide high-resolution spatial information – that is, where in a given sample a particular compound is found -- whereas other configurations scan the bulk sample.
Confocal Raman microscope systems are available from Princeton Instruments, HORIBA, and WITec, among others. Like digital photographs comprised of red, green, and blue channels, the images these systems produce are composites in which each "pixel" is a separate chemical component.
WITec's confocal Raman microscopes can assemble images of 200 x 200 spectral "pixels" in about 40 seconds, says marketing director Harald Fischer. Because the microscopes are "confocal," they can actually collect one such image for each of a series of optical planes (called a "z-stack"), allowing researchers to probe deep beneath a sample surface to build three-dimensional reconstructions.
Trentelman's lab uses a Renishaw Raman microscope system to, among other things, probe an illustration's pigment particles one by one to discern their molecular makeup.
"We wanted to understand the behavior of the individual components," Trentelman explains. "If it was a pure material, that wouldn't be necessary, but works of art are rarely pure materials."
The same could be said of biological tissue. Nevertheless, Anita Mahadevan-Jansen, professor of biomedical engineering and neurological surgery at Vanderbilt University, has devised a system that applies Raman to such clinical problems as the detection of cervical precancerous lesions, characterization of bone mineralization, and margin assessment in breast tumor resection.
"Raman allows you to distinguish subtle changes in tissue," explains Mahadevan-Jansen, whose system is sufficiently small and portable to be used in a clinical exam room or operating theater.
Employing a 785-nm laser from Innovative Photonic Solutions, Kaiser Optical Systems spectrograph, and a pen-sized fiber-optic probe, Mahadevan-Jansen's instrument can, for instance, probe the biochemical signature of cervical lesions simply by touching them in vivo. On-board software then stratifies the growths into normal, low-grade precancerous, high-grade precancerous, or cancerous. In a pre-clinical test of the system on 200 patients, the system exhibited 95% accuracy, she says.
Surface-Enhanced Resonance Raman Scattering (SERRS)
Duncan Graham, Professor of Pure and Applied Chemistry at the University of Strathclyde, Scotland, also uses Raman in a biomedical context. The problem Graham wants to solve is diagnostic multiplexing. Specifically, he is exploiting Raman – or more specifically, surface-enhanced resonance Raman scattering (SERRS) – to develop highly multiplexed DNA-based assays for pathogen identification.
SERRS relies on the fact that metal acts as a sort of amplifier of Raman scattering, increasing the signal by as much as 10e14-fold, Graham says. In this case, Graham couples pathogen-specific DNA probes – one for Chlamydia, say, and one for gonorrhea – to different fluorophores, each of which is also tagged with a silver nanoparticle. That nanoparticle amplifies the natural Raman-scattering potential of the fluorophores, enabling Graham to detect single molecules of pathogen DNA, not by their fluorescence, but by their Raman signatures.
"We use fluorophores, but not as fluorophores. We are making use of another property of them, and that is their scattering," explains Graham, who co-founded D3 Technologies Ltd. to commercialize the technology.
Ahura Scientific (now part of Thermo Fisher Scientific as Portable Optical Analysis) and Ocean Optics address another market niche: on-site detection and identification of unknown chemical compounds. Both companies offer handheld Raman spectrometers for use in the field, for instance by first-responders, quality-control officers, or customs agents.
"The whole idea is the instrument must be self-contained," says Duane Sword, Vice President, Thermo Scientific Portable Optical Analysis (formerly Ahura Scientific). "There is no tether to an instrument." (That distinguishes handheld spectrometers from fiber-optic systems used, for instance, for pharmaceutical manufacturing quality-control, in which the scan head is located some distance from the analyzer itself.)
According to Sword, the company's instruments are designed to answer three questions of unknown compounds: What is it? Is it authentic? And, is it dangerous?
Suppose, for instance, that a customs agent searches a passenger entering the United States. That individual is carrying a bottle of what appears to be mother’s milk. Using a handheld instrument, the agent can scan the contents of the bottle (simply by pointing the nose of the instrument at the translucent container) to determine if the bottle contains a threat. If it is not cleared as a benign substance, the system identifies the compound so authorities know how to appropriately respond to a potentially dangerous liquid. By April, Sword says, Ahura's spectrometers' on-board compound libraries will contain some 10,000 signatures, and users can "train" the device to recognize still others as needed.
"If you see the fingerprint, you can see it again," he says. "It's like taking a photo of a chemical at the molecular level."
When choosing a Raman spectrometer, the key thing to consider, says Whitley, is the sample. "Is it in a bottle? In a process stream? Is it very small? That tells me which sampling optics to recommend."
Next up: the excitation sources. Some samples auto-fluorescence when hit with light of certain wavelengths. "You can get around that by choosing the right excitation wavelength," Whitley says. Biological specimens, for instance, tend to autofluoresce under blue or green excitation, but less so with NIR.
Finally, Whitley asks whether the user will be doing bulk measurements, or is spatial information needed? The answer determines whether a he will recommend a bulk detection or microscopy system.
Other variables to consider include sensitivity (a function of the CCD detector and the optics used to filter out Rayleigh-scattered photons), lateral resolution (for microscopy systems), spectral resolution, and configurability (for instance, can you dial down the laser power so you don't burn precious samples?).
Spectral resolution, given in "wavenumbers," or cm-1, governs the instrument's ability to unambiguously identify unknown compounds. According to Whitley, a high-resolution spectrometer can resolve peaks separated by one or fewer wavenumbers (that is, if its resolution is >1); a medium-resolution instrument has resolution of between one and eight wavenumbers; and a low-resolution instrument has resolution >8 wavenumbers.
Princeton Instruments' Gooding, whose company specializes in both CCD cameras and spectragraphs, advises potential customers especially to consider the system's detector. "You want a high quantum-efficiency detector, which means a back-illuminated CCD camera," he says. A high quantum-efficiency, low-noise, cooled CCD camera, he adds, "will give you up to 95% quantum efficiency, which means 95% of photons incident on that detector are converted to a signal."
Ultimately, though, specification sheets will get you only so far; some samples are tougher to measure than others. So Fischer suggests taking the instrument for a spin. "Test a standard sample on a sample instrument," he says. "Then [users] can compare very quickly which gives the best results in x and y, or x and z, or which acquires spectra very quickly with [their] samples."
Please check out our Raman Spectroscopy section for more information or to find manufacturers that sell these products.