Featured Article
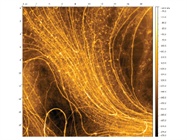
As a once complex and expensive technique becomes simpler and less expensive, it finds more applications in basic and applied research
As scientists, we strive to see more, to look more deeply into nature and to do so at higher resolution. Atomic force microscopy (AFM) provides one way to do that with resolution at the nanoscale. AFM moves a nano-size tip over a sample’s surface, and measures a variety of features, such as height, friction and magnetism. The lateral resolution might be only about 30 nm, but the vertical resolution can reach 0.1 nm. “During the early stages of AFM instruments—from approximately 1990 through 2010—AFMs were used primarily by experts whose primary objective was to demonstrate how these unique instruments could be used,” says Paul West, founder of AFMWorkshop in Signal Hill, Calif. “What’s most interesting to me is that recently AFMs have become routine tools used in support of research and development projects and may be operated by scientists and engineers who are not AFM experts.”
Custom manufacturing requirements initially drove up the cost of an AFM. “Over the past five years,” says West, “new components manufactured for other advanced technology products have become more widely available, and this has resulted in a cost reduction for the manufacturing of AFMs and has thus significantly reduced customer prices for AFMs.” He points out, for example, that the price of an xyz piezoelectric scanner with linearizers, an important component in an AFM, has fallen from $18,000 to $4000, and that the overall price of a high-quality instrument has dropped from $300,000 to less than $50,000.
Improvements in ease of use also make AFM attractive to more users. West explains, “An organic chemist or materials scientist with no prior knowledge of an AFM instrument can now afford to purchase a unit, and to use it daily in support of their research by imaging materials fabricated in their lab.”
Wide capabilities
Modern AFMs also work better and provide more information than early models. The technique delivers high spatial and temporal resolution, and dynamic events can be recorded on the nanoscale in milliseconds. “This [instrument] can analyze, say, a phase transition in an inorganic crystal or complex material,” says Thomas Mueller, director of AFM development applications at Bruker Nano Surfaces Division in Santa Barbara, Calif.
Atomic force microscopy reveals fine details of samples, like the self-assembled lipid nanotubes shown here. (Image courtesy of AFMWorkshop.)The first AFMs used a contact mode with the probe’s tip touching the sample, much like a phonograph needle in contact with a record. Like a needle scratching a record, the contact mode can damage a sample. So scientists developed a tapping mode, in which the probe’s tip oscillates up and down—literally tapping the sample’s surface, which does less damage.
Scientists kept developing new modes of AFM. “There have been 100 or so modes, but none after contact and tapping became the next big thing,” says Mueller. That changed, he says, with Bruker’s PeakForce Tapping. With this mode, a scientist can control the interaction between the AFM tip and the sample instantly and linearly, keeping the force down to piconewtons. “We came up with PeakForce Tapping just a few years ago,” Mueller says, and applications have already been described in more than 1000 articles, including ones in Science and Nature.
Scanning probe microscopy requires software such as SPIP software to process the data to make and analyze images. (Image courtesy of Image Metrology.)The range of measurements that scientists can make with AFM explains its growing popularity. “With PeakForce Tapping, you can go atom by atom to map out stiffness, sample deformation, density changes and such at the surface,” says Mueller. In addition, other measurements can be added to nanomechanical information provided by the AFM. Bruker’s Inspire combines AFM with infrared microscopy, which can create a chemical map of a sample with 10-nm resolution. Mueller says, “We see a strong interest in combining AFM with other modes to get multimodal data at the nanoscale.”
How the probe scans the sample also matters. Some platforms move the sample, but that can slow down or prevent imaging of heavier samples. So Bruker developed FastScan, which moves the tip over the sample. “This makes faster scanning more routine,” Mueller explains, “so you can capture dynamics with it, for any sample.”
Seeing in 2-D
Some scientists use AFM to study two-dimensional materials, which are single-atom layers. Mueller says that this work really took off after Andre Geim and Konstantin Novoselov of the University of Manchester in the U.K. received the 2010 Nobel Prize in Physics for work on single-atom layers of graphene, which is composed of carbon atoms. “More recently this has grown into wider research in 2-D materials,” Mueller says.
In Nature Physics in 2014, Geim, Novoselov and their colleagues described the behavior of graphene layered on hexagonal boron nitride. “Using PeakForce QNM for very high-resolution nano-mechanical mapping at the Angstrom scale, they mapped the stress–strain relief in the material, uncovering a transition to commensurability upon lattice alignment,” Mueller says. “They could not have done this with any other technology.”
Graphene is strong and an efficient conductor of heat and electricity. It can be used in various electronics assemblies, including batteries and semiconductors, and in composite materials; understanding its behavior allows scientists to fine-tune graphene in different applications.
Software for processing AFM data
AFM is part of a broader class of imaging called scanning probe microscopy (SPM), and all of these techniques require software to process the data and create accurate images. “It is not uncommon that the images generated by SPMs have imperfections due to instrument inaccuracies or due to interference from the surrounding environment—for example, temperature variances,” says Jan F. Jørgensen, CEO at Image Metrology in Hørsholm, Denmark. “Therefore, it is important to have powerful image software that can determine and correct for these imperfections and create more precise measuring results than the SPM instrument can do on its own.”
A software package for SPM should provide several features. “Visualization software is a part of any SPM solution as it is essential for the production of images,” says Jørgensen. “But in addition to that, advanced image processing software, for exploring, correcting, measuring and analyzing complex data is needed for almost any professional and academic SPM user.” He adds, “What the user gets is increased accuracy and efficient analysis.”
For such needs, Image Metrology developed SPIP software, which processes a variety of nano- and microscale data, especially from SPM. “One of the challenges with SPM software is that there are many equipment suppliers and not one common file format for SPM images,” Jørgensen explains. “SPIP opens almost any file format and enables very detailed and accurate analysis by a comprehensive set of tools.” He adds, “Even for advanced functions, SPIP has automation facilities and predefined formulas that can easily be adjusted to fit individual needs.”
AFM for medicine
Today’s simpler and more robust AFM is also finding clinical applications. Researchers at Purdue University (West Lafayette, Ind.) used high-speed AFM to image breast cancer cells and molecular events that help the cancer spread. They showed that inhibiting a particular protein—spleen tyrosine kinase—lets the cancerous cells spread, and the AFM showed that this inhibition caused changes in the shape of the cancer cells. This kind of technology could be used to study the effect on cancer drugs on specific physical features of cancerous cells.
AFM can also be used to study infectious diseases. Belgian researchers Philippe Herman-Bausier and Yves Defrêne used AFM to study the molecular binding of Staphylococcus epidermidis, as reported in the October 20, 2015, issue of Molecular Microbiology. This information could help scientists battle infections caused by this microorganism.
As AFM gets even easier to use and more economical, applications in both basic and applied research will continue to emerge. The technique holds promise in the field of medicine, and can quickly and accurately describe the physical properties of a sample at the atomic level.
Mike May is a freelance writer and editor living in Ohio. He can be reached at [email protected].